In sports, as in science, there are two very different types of heroes. There are the giants who lead the their teams and their sport, winning championships and accolades, for years, and whose fame lives on for decades: the Michael Jordans, the Peles, the Lou Gherigs, the Joe Montanas. And then there are the unlikely heroes, the ones who just happen to have a really good day at a really opportune time; the substitute player who comes on the field for an injured teammate and scores the winning goal in a championship; the fellow who never hits a home run except on the day it counts; the mediocre receiver who turns a short pass into a long touchdown during the Super Bowl. We celebrate both types, in awe of the great ones, and in amused pleasure at the inspiring stories of the unlikely ones.
In science we have giants like Newton, Darwin, Boyle, Galileo… The last few decades of particle physics brought us a few, such as Richard Feynman and Ken Wilson, and others we’ll meet today. Many of these giants received Nobel Prizes. But then we have the gentlemen behind what is commonly known as the Higgs particle — the little ripple in the Higgs field, a special field whose presence and properties assure that many of the elementary particles of nature have mass, and without which ordinary matter, and we ourselves, could not exist. Following discovery of this particle last year, and confirmation that it is indeed a Higgs particle, two of them, Francois Englert and Peter Higgs, have been awarded the 2013 Nobel Prize in physics. Had he lived to see the day, Robert Brout would have been the third.
My articles Why The Higgs Particle Matters and The Higgs FAQ 2.0; the particles of nature and what they would be like if the Higgs field were turned off; link to video of my public talk entitled The Quest for the Higgs Boson; post about why Higgs et al. didn’t win the 2012 Nobel prize, and about how physicists became convinced since then that the newly discovered particle is really a Higgs particle;
The paper written by Brout and Englert; the two papers written by Higgs; the paper written by Gerald Guralnik, Tom Kibble and Carl Hagen; these tiny little documents, a grand total of five and one half printed pages — these were game-winning singles in the bottom of the 9th, soft goals scored with a minute to play, Hail-Mary passes by backup quarterbacks — crucial turning-point papers written by people you would not necessarily have expected to find at the center of things. Brout, Englert, Higgs, Guralnik, Kibble and Hagen are (or rather, in Brout’s case, sadly, were) very fine scientists, intelligent and creative and clever, and their papers, written in 1964 when they were young men, are imperfect but pretty gems. They were lucky: very smart but not extraordinary physicists who just happened to write the right paper at the right time. In each case, they did so
- …not by studying the real world but by studying simplified, imaginary worlds, in which it was easier to learn the general lessons they wanted to extract…
- ...with a motivation based on a real-world question — the origin of mass for hadrons — for which, it turns out, their ideas do not apply… [Hadrons, including protons and neutrons, are today known to be particles made from quarks and gluons; but that wasn’t known in the early 1960s.]
- …and with no suspicion that their results would instead be applied to a different real-world question: the possibility that the weak nuclear force is associated with photon-like particles, called W and Z, that have a mass. [A photon is a particle of light, has spin=1, and, unlike the W and Z particles, has no mass.]
History in general, and history of science in particular, is always vastly more complex than the simple stories we tell ourselves and our descendants. Making history understandable in a few pages always requires erasing complexities and subtleties that are crucial for making sense of the past. Today, all across the press, there are articles explaining incorrectly what Higgs and the others did and why they did it and what it meant at the time and what it means now. I am afraid I have a few over-simplified articles of my own. But today I’d like to give you a little sense of the complexities, to the extent that I, who wasn’t even alive at the time, can understand them. And also, I want to convey a few important lessons that I think the Hi(gg)story can teach both experts and non-experts. Here are a couple to think about as you read:
1. It is important for theoretical physicists, and others who make mathematical equations that might describe the world, to study and learn from imaginary worlds, especially simple ones. That is because
- 1a. one can often infer general lessons more easily from simple worlds than from the (often more complicated) real one, and
- 1b. sometimes an aspect of an imaginary world will turn out to be more real than you expected!
2. One must not assume that research motivated by a particular goal depends upon the achievement of that goal; even if the original goal proves illusory, the results of the research may prove useful or even essential in a completely different arena.
My summary today is based on a reading of the papers themselves, on comments by John Iliopoulos, and on a conversation with Englert, and on reading and hearing Higgs’ own description of the episode.
The story is incompletely but perhaps usefully illustrated in the figure below, which shows a cartoon of how four important scientific stories of the late 1950s and early 1960s came together. They are:
- How do superconductors (materials that carry electricity without generating heat) really work?
- How does the proton get its mass, and why are pions (the lightest hadrons) so much lighter than protons?
- Why do hadrons behave the way they do; specifically, as suggested by J.J. Sakurai (who died rather young, and after whom a famous prize is named), why are there photon-like hadrons, called rho mesons, that have mass?
- How does the weak nuclear force work? Specifically, as suggested by Schwinger and developed further by his student Glashow, might it involve photon-like particles (now called W and Z) with mass?
These four questions converged on a question of principle: “how can mass be given to particles?”, and the first, third and fourth were all related to the specific question of “how can mass be given to photon-like particles?’’ This is where the story really begins. [Almost everyone in the story is a giant with a Nobel Prize, indicated with a parenthetic (NPyear).]
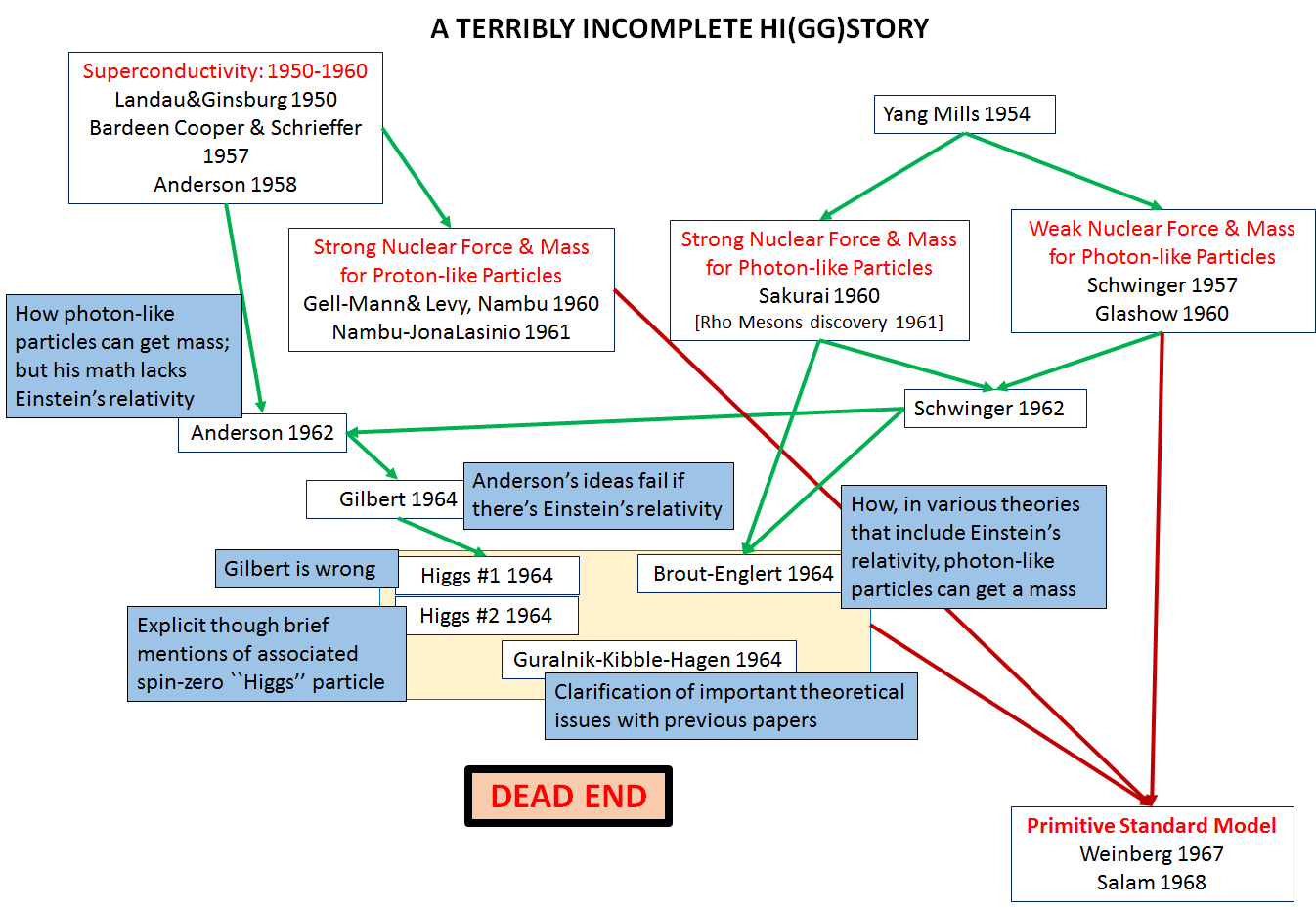
In 1962, Philip Anderson (NP1977), an expert on (among other things) superconductors, responded to suggestions and questions of Julian Schwinger (NP1965) on the topic of photon-like particles with mass, pointing out that a photon actually gets a mass inside a superconductor, due to what we today would identify as a sort of “Higgs-type’’ field made from pairs of electrons. And he speculated, without showing it mathematically, that very similar ideas could apply to empty space, where Einstein’s relativity principles hold true, and that this could allow elementary photon-like particles in empty space to have mass, if in fact there were a kind of Higgs-type field in empty space.
In all its essential elements, he had the right idea. But since he didn’t put math behind his speculation, not everyone believed him. In fact, in 1964 Walter Gilbert (NP1980 for chemistry, due to work relevant in molecular biology — how’s that for a twist?) even gave a proof that Anderson’s idea couldn’t work in empty space!
But Higgs immediately responded, arguing that Gilbert’s proof had an important loophole, and that photon-like particles could indeed get a mass in empty space.
Meanwhile, about a month earlier than Higgs, and not specifically responding to Anderson and Gilbert, Brout and Englert wrote a paper showing how to get mass for photon-like particles in empty space. They showed this in several types of imaginary worlds, using techniques that were different from Higgs’ and were correct though perhaps not entirely complete.
A second paper by Higgs, written before he was aware of Brout and Englert’s work, gave a simple example, again in an imaginary world, that made all of this much easier to understand… though his example wasn’t perhaps entirely convincing, because he didn’t show much detail. His paper was followed by important theoretical clarifications from Guralnik, Hagen and Kibble that assured that the Brout-Englert and Higgs papers were actually right. The combination of these papers settled the issue, from our modern perspective.
And in the middle of this, as an afterthought added to his second paper only after it was rejected by a journal, Higgs was the first person to mention something that was, for him and the others, almost beside the point — that in the Anderson-Brout-Englert-Higgs-Guralnik-Hagen-Kibble story for how photon-like particles get a mass, there will also generally be a spin-zero particle with a mass: a ripple in the Higgs-type field, which today we call a Higgs-type particle. Not that he said very much! He noted that spin-one (i.e. photon-like) and spin-zero particles would come in unusual combinations. (You have to be an expert to even figure out why that counts as predicting a Higgs-type particle!) Also he wrote the equation that describes how and why the Higgs-type particle arises, and noted how to calculate the particle’s mass from other quantities. But that was it. There was nothing about how the particle would behave, or how to discover it in the imaginary worlds that he was considering; direct application to experiment, even in an imaginary world, wasn’t his priority in these papers.
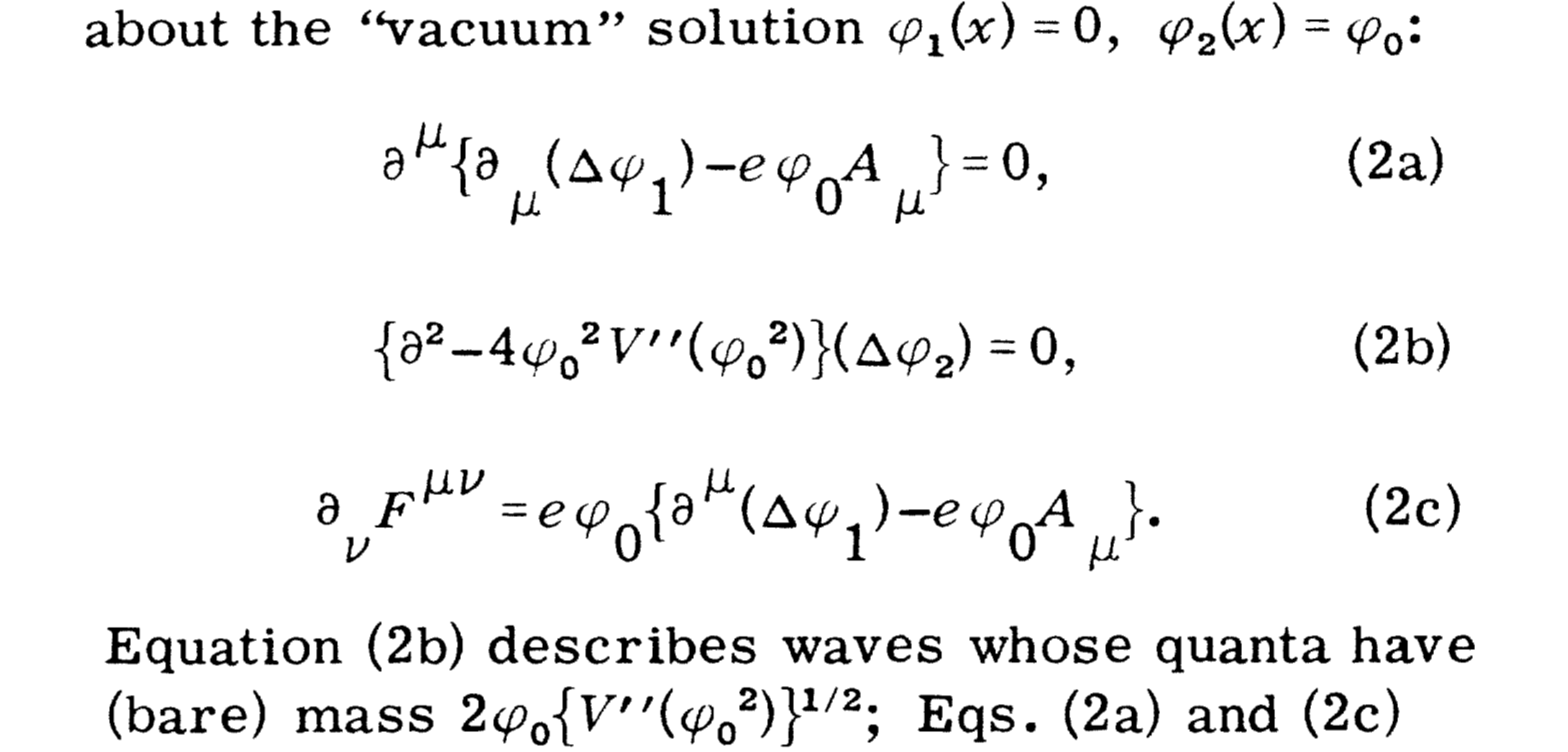
About the “Higgs-type” particle, Anderson says nothing; Brout and Englert say nothing; Guralnik et al. say something very brief that’s irrelevant in any imaginable real-world application. Why the silence? Perhaps because it was too obvious to be worth mentioning? When what you’re doing is pointing out something really “important’’ — that photon-like particles can have a mass after all — the spin-zero particle’s existence is so obvious but so irrelevant to your goal that it hardly deserves comment. And that’s indeed why Higgs added it only as an afterthought, to make the paper a bit less abstract and a bit easier for a journal to publish. None of them could have imagined the hoopla and public excitement that, five decades later, would surround the attempt to discover a particle of this type, whose specific form in the real world none of them wrote down.
In the minds of these authors, any near-term application of their ideas would probably be to hadrons, perhaps specifically Sakurai’s theory of hadrons, which in 1960 predicted the “rho mesons”, which are photon-like hadrons with mass, and had been discovered in 1961. Anderson, Brout-Englert and Higgs specifically mention hadrons at certain moments. But none of them actually considered the real hadrons of nature, as they were just trying to make points of principle; and in any case, the ideas that they developed did not apply to hadrons at all. (Well, actually, that’s not quite true, but the connection is too roundabout to discuss here.) Sakurai’s ideas had an element of truth, but fundamentally led to a dead end. The rho mesons get their mass in another way.
Meanwhile, none of these people wrote down anything resembling the Higgs field which we know today — the one that is crucial for our very existence — so they certainly didn’t directly predict the Higgs particle that was discovered in 2012. It was Steven Weinberg (NP1979) in 1967, and Abdus Salam (NP1979) in 1968, who did that. (And it was Weinberg who stuck Higgs’ name on the field and particle, so that everyone else was forgotten.) These giants combined
- the ideas of Higgs and the others about how to give mass to photon-like particles using a Higgs-type field, with its Higgs-type particle as a consequence…
- …with the 1960 work of Sheldon Glashow (NP1979), Schwinger’s student, who like Schwinger proposed the weak nuclear force was due to photon-like particles with mass,…
- …and with the 1960-1961 work of Murray Gell-Man (NP1969) and Maurice Levy and of Yoichiro Nambu (NP2008) and Giovanni Jona-Lasinio, who showed how proton-like or electron-like particles could get mass from what we’d now call Higgs-type fields.
This combination gave the first modern quantum field theory of particle physics: a set of equations that describe the weak nuclear and electromagnetic forces, and show how the Higgs field can give the W and Z particles and the electron their masses. It is the primitive core of what today we call the Standard Model of particle physics. Not that anyone took this theory seriously, even Weinberg. Most people thought quantum field theories of this type were mathematically inconsistent — until in 1971 Gerard ‘t Hooft (NP1999) proved they were consistent after all.
The Hi(gg)story is populated with giants. I’m afraid my attempt to tell the story has giant holes to match. But as far as the Higgs particle that was discovered last year at the Large Hadron Collider, the unlikely heroes of the story are the relatively ordinary scientists who slipped in between the giants and actually scored the goals.
70 Responses
Just want to say your article is as surprising. The clarity in your post is simply spectacular and i could assume you are an expert on this subject.
Well with your permission allow me to grab your feed to keep updated with forthcoming post.
Thanks a million and please continue the rewarding work.
Hello are using WordPress forr your site platform?
I’m new tto tthe blog world but I’m trying to get started and create my own.Do you need
any html coding expertise to make your own blog? Any helpp would be really appreciated!
Good day! This post couldn’t be written any better! Reading through this post reminds
me of my old room mate! He always kept chatting about
this. I will forward this write-up to him. Pretty sure he will have
a good read. Thank you for sharing!
In fact when someone doesn’t be aware of afterward its up to
other viewers that they will help, so here it occurs.
Great site you’ve got here.. It’s hard to find
high-quality writing like yours nowadays. I honestly appreciate people like you!
Take care!!
This is a topic which is close to my heart…
Cheers! Exactly where are your contact details though?
Thanks for this article! 🙂
The equation (2) are derived from the Lagrangian density, that is the same in all reference frames. It is a Lorentz scalar. This makes the Lagrangian density a fundamental quantity in quantum field theory. The Standard Model of physics is based on the Lagrangian density which in quantum physics is generally called just the Lagrangian, without the density.
Excuse me for my insistence, but please try to explain these concepts without oversimplification … 😉 thanks again
Electro-weak unification of QFT through Higgs field had precipitated (computation) the “Higgs particle” ?
“You have to be an expert to even figure out why that counts as predicting a Higgs-type particle”
MASS ENERGY PRODUCES HEAT – so in super conductivity photons acquire “relative mass” and behave like electrons – but unlike mass energy produces no heat ?
But gluon (string?) out of vacuum energy produces no heat and electro magnetic radiation.
Relativity explaining the unexplained, is important rather than its explanation of observable and measurable (at rest mass) ?
I’m not clear what you’re saying or asking, veeramohan, but as for relativity, see Einstein’s E=mc² paper. He used L instead of E, but nevermind. Note “the mass of a body is a measure of its energy-content”. Also note “if a body gives off the energy L in the form of radiation, its mass diminishes by L/c²”. If you trap a massless photon in a mirror-box you add mass to that system. If you then open the box, it’s a radiating body that loses mass. And “radiation conveys inertia between the emitting and absorbing bodies”.
Mr duffieldjohn,
what I understand from the below link in German is…
What Higgs predicted was the mass of massless Gluons. But Weinberg used this “Higgs mechanism” for uniting electromagnetic and weak forces (electroweak).
Loss of mass through radiation is radioactive decay (weak force). Both strong force and weakforce have short range – and “massless” gauge bosons acquire mass thru higgs mechanism. But there is no prediction that gluons get their mass from Higgs mechanism – Gluons are massless. Why photons didn’t interact with higgs mechanism ?
“Radiation conveys inertia between the emitting and absorbing bodies”. THIS WILL NOT APPLY TO “RELATIVE MASS” LIKE GLUONS – PHOTONS ARE EXCEPTIONAL – because it EXPLICIT PARTICLE NATURE (rest mass).
If you have a massless Dirac particle there are excited states of positive energy and there’s the sea, and there is no gap between them, but if you have a massive particle then those are split apart. There is an energy difference between the sea and the states of the fermions, so there is a BCS-like gap of 2mc2.
This is basically the way in which Nambu’s BCS model involving spontaneous symmetry breaking generates fermion masses – so in super conductivity photons acquire “relative mass” and behave like electrons.
Philip Anderson pointed out that in a superconductor the Goldstone mode becomes a massive plasmon mode, due to its electromagnetic interaction.
THE PARTICLE FOUND IN CERN MUST HAVE the “REST MASS”.
Wofür Higgs – sicherlich zu recht – den Nobelpreis bekommen hat, ist die Vorhersage des prinzipiellen Mechanismus, mit dem Austauschteilchen (GLUONS) eine Masse bekommen können, aber das am CERN gefundene Teilchen hat er nicht vorhergesagt.
Out of curiosity: why was Englert the first author of the Englert-Brout paper? didn’t they follow the usual alphabetical order at that time?
From the article it seems that the first team to suggest something like the “Higgs field” were Murray Gell-Man et al. in 1961 (even before Anderson) for fermions. Is that correct?
I have noticed that some readers on this blog have expressed sorrow at Anderson and experimental physicists not receiving share of this Nobel Prize. Surprisingly no one has mentioned unfairness of Guralnik, Hagen and Kibble not receiving any part of it. Matt has indeed mentioned their names in this context. I understand Weinberg has also said that they deserved the Nobel Prize. It is well known that they came up with the same model independently and were only late by a few weeks in publishing. I recently read a historical review by Guralnik (http://arxiv.org/abs/0907.3466v1). I believe their story and looks like they lost out only because of three person maximum rule of Nobel committee. Here are some quotes from this article “Shortly thereafter, as we were literally placing the manuscript in the envelope to be sent to PRL, Kibble came into the office bearing two papers by Higgs and the one by Englert and Brout. These had just arrived in the then very slow and unreliable (because of strikes and the peculiarities of Imperial College mail”)…. ” After seeing the competing EBH analyses, we unhesitatingly thought that we should do the completely honest thing and reference them, as they were clearly relevant with examples, even if not convincing to us. Our paper was finished and typed in final form when we saw these other works and made this decision. We only altered the manuscript by adding in several places references to these just-revealed papers. Not a single thought or calculation was removed or added, nor was any change made but to the referencing in our manuscript as the result of Kibble’s having pointed out the existence of these new papers. In retrospect, I wish we had added the true statement — “after this paper was completed, related work by EB and H was brought to our attention.” “We were naive enough to feel that these other articles offered no threat to our insights.” Sorry that is life!
From:
The Nobel Prize in Physics 2008- Presentation Speech by Lars Brink
“…How is this reflected in the fundamental laws of nature, the ones
that govern physics at the smallest distances? When elementary
particles and the forces that act between them were studied in
experiments from the 1950s onward, it was found that everything that
the underlying symmetries allow can happen. Symmetries must therefore
impose enormously strong restrictions for the laws to be meaningful.
How can this lead to our universe, with its four kinds of fundamental
forces and great variety of elementary particles? The decisive idea
came from Yoichiro Nambu, who in 1960 showed that the fundamental
laws of nature can exhibit spontaneous broken symmetries, as
illustrated by the person falling off the Matterhorn. There can be
strong restrictions from large symmetries that are not seen directly
in physical experiments, at least at the energies that can be
produced in today’s large particle accelerators. The symmetries are
there as a consequence of the fundamental laws of physics, but for a
system in its ground state there are no direct signs of the
restrictions that symmetry should impose. This means, for instance,
that the electromagnetic force and the weak nuclear force do not
appear related, even though the underlying theory says so. This is
just one example of Nambu’s ideas that permeate all of modern physics
and have been tested in numerous experiments…”
————————————————–
For one of the papers that the award referenced see:
“Quasi-Particles and Gauge Invariance in the Theory of Superconductivity”
Yoichiro Nambu
Physical Review 117 (1960) p. 648-663
—————————————————
Quick question (a bit off-topic): since you mentioned John Iliopoulos’ name, shouldn’t the GIM mechanism receive a similar recognition, before it’s too “late”, or is Glashow’s Nobel prize a showstopper?
Why not call it the BHE boson?
The “H” boson is probably as far as we will get from Higgs’ name.
There appeared to be a push to call it a H-boson a year back but it didn’t catch on. I guess Higgs has a nice ring to it. Shame that dyson didn’t predict a new particle, feels like an ideal name.
Dyson believed in bare particles. Maybe all bare particles can be called Dyson’ ones?
I appreciate the points you’re making in this article but it seems a bit of a slip in decorum, on today of all days, to voice the comparison to a mediocre receiver who happens to score a touchdown.
Perhaps. But I would be more than glad to be a mediocre receiver who scores such a touchdown.
This really is a great time to be alive and be a scientist of any stripe. Delusional myths are being relegated to the historical trash bin or convenient explanations for what many are too lazy to consider and accept.
Congratulation to Higgs, Englert for the Nobel and all the others ( Brout ,Gerald Guralnik, Tom Kibble , Carl Hagen and Philip Anderson) who played a part in this Higgs story. This Higgs history carries so much *spirits*, and even *Nature* itself must give a salute regardless of ….
Now, the Higgs game is over, and we can move forward, to new mysteries of Nature, the dark mass and dark energy.
I predict that those two are resolved during next 12 months. What then? 🙂
Can you provide a link to all of the papers that you mentioned?
Wikipedia for “Higgs boson” has them all.
Let me be more precise then: the discovery mode of the Higgs boson was by decay to two vector bosons (as the virtual decays are written h ->WW* or h ->ZZ*, I think that is reasonable language even if slightly inaccurate).
Higgs computed the decay of the scalar to vector bosons in a fictitious theory that was not the Standard Model. This amplitude is *not* the amplitude for the SM Higgs to decay (sorry if this appeared to be what I was saying). However, it *is* the cognate amplitude in the fictitious theory to the h -> ZZ* and h -> WW* decays in the Standard Model.
It is true that some comment on Higgs’ 1966 paper would have been nice. I had to choose between clarity, accuracy and brevity, so I decided to focus on the 1964 papers; the 1966 paper is clearly *not* the reason Higgs was awarded a prize.
I generally agree with what you say – but you are being unfair to Higgs in one very important way. Higgs *did* further discuss the behaviour of the heavy scalar, and in particular computed the amplitude for it to decay to two vectors bosons, in his 1966 paper (see http://prola.aps.org/pdf/PR/v145/i4/p1156_1)
And this of course was the discovery mode of the Higgs boson.
That is incorrect, actually. He computed the amplitude for it to decay to two vector bosons TO WHICH IT HAD GIVEN MASS. In fact, the discovery modes were (1) to two photons (which don’t have mass) which the Higgs interacts with only via a quantum effect, and (2) to one Z particle and one virtual Z particle. Higgs didn’t do either computation. More to the point, he did the calculation in an imaginary world. This is not to take anything away from Higgs; he didn’t know Glashow’s work and so it never occurred to him to study that theory. Which, for him, is quite a shame; he might have invented Weinberg’s theory before Weinberg.
Really nice summary here, and of course it ties in nicely with your recent posts.
Reading this post was a pleasant review session for me, since I read Frank Close’s book The Infinity Puzzle (hope those italics work) almost two years ago now, shortly after its publication in late 2011. Close interweaves the Hi(gg)story itself with intriguing biographical accounts of the protagonists. It’s a good read, and I’d recommend it to anyone here who’s interested in the long version of this post.
That sounds interesting. Frank Close is mentioned here referring to a “relativistic aether”. That would make the Higgs field responsible for photon momentum as well as electron mass. More “cosmic elastic” than “cosmic treacle”, but it fits with E=mc² and would mean the Higgs field is responsible for 100% of the mass of matter rather than 1%.
Is it possible that a spin 0 particle twists and turns?
nice.
Marten: No. But when you make a spin 1 particle twist and turn, via say gamma-gamma pair production, you call it a spin ½ particle. Momentum is resistance to change-in-motion for a wave on an open path, mass is resistance to change-in-motion for a wave in a closed path.
Thank you for telling me.
Anderson was unfaily left out of the picture in this year Nobel award. And so was Cabbibo, few years ago. One has many reasons to doubt the fairness of the Nobel enterprise in science…
Human affairs are based on judgment, and they are never entirely fair. It is important to keep both a list of those who won the award and those who were just outside the circle. Dyson, Cabbibo, Bahcall,… there’s a long list. But don’t cry for Anderson. He won a Nobel Prize already.
I am not crying for Anderson. I am only questioning the objectivity of the Nobel committee when it comes to politically motivated reasons. How would you personally feel if you were one of the authors of the Anderson-Higgs mechanism and told that you cannot get the Nobel just because you already won?
Well, if you are the one who calls it the Anderson-Higgs mechanism…..
I am not the only one calling the mechanism Anderson-Higgs. There are many reputable references doing just that, for a good reason.
I can’t speak for Anderson, but if I already had a Nobel prize, I would be happy to cede the limelight to others. How much recognition does a person need in life?! Einstein only got one Nobel, and deserved three. So what? As for the name of the mechanism, leaving off Brout and Englert isn’t fair, since they wrote it down before Higgs did. So… be fair and include them all, or accept the unfairness of history.
It seems so easy to be casual about not receiving the recognition if you are not in that person’s shoes.
“So… be fair and include them all, or accept the unfairness of history.”
If the award winning process was and continues to be unfair, why not get rid of it altogether?
Einstein actually deserved 6 Nobel prices:
Brownian motion: first time clear atoms really exist
Photo-electric effect
Stimulated emission: lasers
Bose-Einstein statistics
Special relativity
General relativity
I think prizes should never be taken too seriously as a distinction of particular people. Prizes and ceremonies are ways of humanity to celebrate itself and the human endeavor, they are ways to express what a community considers valuable. The most important thing about the Nobel prize is not who gets it, but how it raises the appreciation of science and inspires future generations of scientists. Millions dreaming of getting a Nobel prize are more important than one person getting it.
On a personal level, prizes will never be fair. The same goes for naming in science and math. The list of mathematical theorems that are named after someone else than the person who found them is endless, for example. The best thing we can do to honor the unsung heroes is to learn about the many topics they worked on and to appreciate how many people were really involved.
“I think prizes should never be taken too seriously as a distinction of particular people.”
In our real world the winner takes it all. Sad but true.
It would have been hilarious to give it to Anderson — who, if he had had his way, would have ensured that the Higgs would never have been discovered.
Right — that is amusing, indeed. And perhaps there are no accidents here.
I am ignorant of the history here, but why do you say Anderson would do that if he had had his way?
Anderson fought very hard to shut down the effort to build the Superconducting Supercollider in the United States. Had that machine been built, instead of being cancelled after considerable expenditure, the Higgs particle would have been discovered several years earlier and in the United States. Arguably no one has done more harm to particle physics than Anderson.
Before placing such serious blame on Anderson, can you detail for us his motivation against SSC? Also, it is your belief that someone who has contributed heavily to the foundations of the Higgs mechanism deserves to be punished for expressing a different opinion than the mainstream?
Perhaps it is worth mentioning that one school in Bristol, England, now has two Nobel Physics Laureates to its credit.
This is Cotham.School, (formerly known as Cotham Grammar School) attended by both Paul Dirac and Peter Higgs.
What are they doing right?
David Perkin.
Maybe. 2 is nice. How is 8? plus 6 Pulitzers and various National Medals of Science: http://alumni.bxscience.edu/?page=notablealumni
Great post. Concerning Abdus Salam I remember reading about his (lack of) participation in the work that eventually gave him the Nobel prize. Here you have the preprint…
http://arxiv.org/abs/1109.1972
it looks serious work, but who knows…
The version I heard was that P.T. Mathews (then Professor of Theoretical Physics at Imperial College, London) saw a preprint of Weinberg’s paper and walked down the corridor to Abdus Salam’s office and advised him to publish his work as quickly as possible. Salam then wrote it up as far as he had got and presented it at a Conference. This version says that Salam was unaware of Weinberg’s work.
No doubt there are other versions. I certainly don’t guarantee the authenticity of the story, which came to me through coffee-lounge rumour.
David Perkin.
That kind of thing is not unusual; people rush to finish papers, after hearing of competing work, all the time.
Well, according to Norman Dombey, Abdus Salam published his work in the proceedings of a lecture given at a Nobel Symposium held in 1968, when Weinberg’s work was already well-known. Salam claimed that the lecture at the Symposium to be his lecture at Imperial the previous
autumn. That way he could claim he did the work independently of Weinberg. However, no written record of the lecture at Imperial exist.
Arg being dumb. Took me a minute to understand Hi(gg)story.
Why LHC/CERN has not been awarded (although has been mentioned)? It is unfair, to say the least!
The theory is one thing; the experiment is another. Glashow Weinberg and Salam won a prize for predicting the W and Z particles; Carlo Rubbia and Simon van der Meer won a separate prize for discovering them.
Still, there may be many different theories that are compliant with experiments, but the experimental facts are “unique”. Of course, nowadays the experimental results “are seen” via a prism of (an imposed) theory and it looks as it follows the theory.
Carlo Rubbia.
Thanks; fixed