Over the past 130 years, physicists have discovered that pretty much everything material, including rocks and rain, sun and sunshine, ocean waves and radio waves, can be described in terms of particles (and their corresponding fields.) Experiments have uncovered a large handful of types of particles that appear so far to be elementary (i.e., not made from yet more elementary things.) The full complexity of our daily world is constructed from just a few of these. The rest of the particles are evanescent, decaying away so quickly that we don’t encounter them in normal circumstances. But they may hold the keys to secrets of the universe that continue to elude us at the moment.
In this article you will find a brief overview of our present state of knowledge, as of July 2022, showing you the particles we know about and how they can be organized usefully into a few classes… a sort of periodic table of the particles, with a few twists. Along the way you’ll learn what the Higgs field does to the various particles, and the crucial role it plays in our universe.
Our present knowledge, along with our simplest conjecture for the workings of the Higgs particle and field, is summarized in a set of equations called “The Standard Model of Particle Physics”, or just “Standard Model” for short. The elementary particles of the Standard Model have somewhat wacky names (for historical reasons) and a very wide range of masses. (All masses in this page and throughout this site are “rest masses”.) In Figure 1 below, notice that
- I’ve drawn heavier particles at the top, the lighter particles at the bottom. (I do this because massless is as low as a particle can go, but particles can have an arbitrarily high mass; in short, there’s a hard floor below, but above, the sky’s the limit.)
- Instead of masses I’ve given the equivalent mass-energies (E = m c-squared) which is what particle physicists typically use. (Keeping track of energy, which is never lost or gained, is easier than keeping track of mass, which can change in some processes, such as decays.) The unit of a GeV is about the mass-energy of the lightest atom, hydrogen.
- I’ve indicated three classes of particles — charged leptons (blue disks), the neutrinos (black disks), and the quarks (red disks). (The quarks are typically sub-divided into two classes, up-type and down-type, which differ only in their electric charge.) The importance of this classification will become clear later.
- I have indicated three forces in boxes, along with their force carrier particles (see below). (Physicists call the forces “interactions”, and the force carriers “mediators”; I am using more intuitive language here.) I have not indicated a fourth force, that of gravity, to avoid clutter on the picture.
- The Higgs field (or something playing its role) is known to be on average non-zero in nature. I’ve represented this with a great green sea. (You can learn more about fields and particles, and the Higgs in particular, in the video clips from my public talk at the Secret Science Club.)
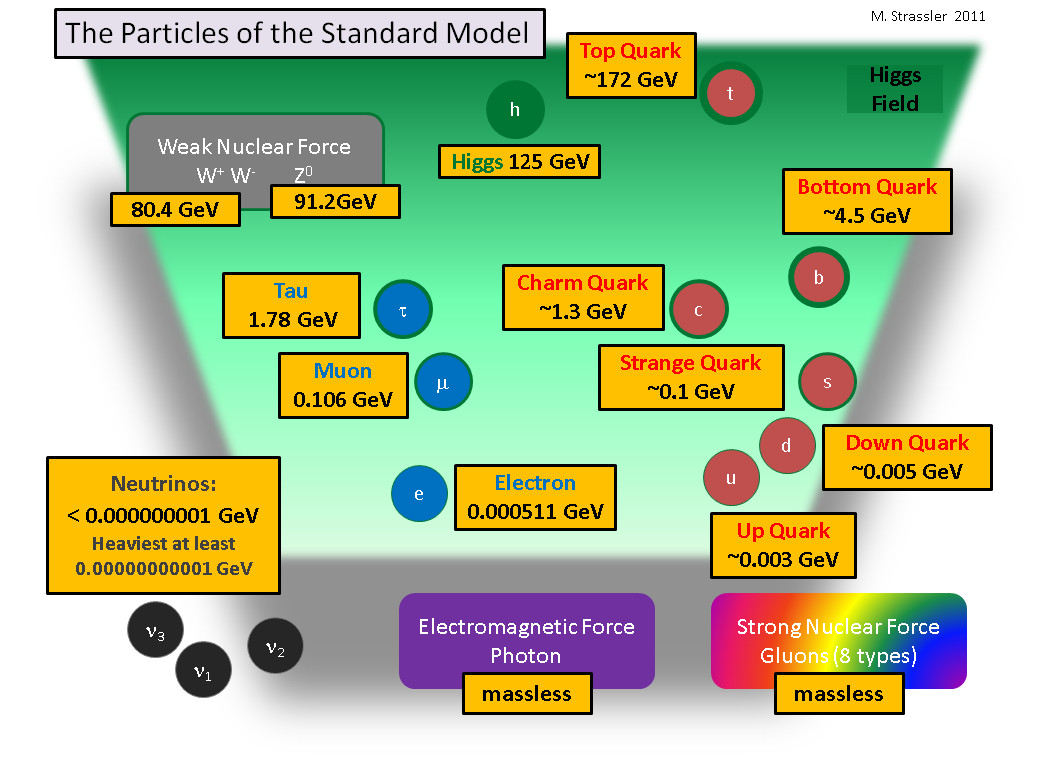
What are all these particles? [All of these particles have anti-particles, but to keep things short I won’t mention them, except to point you to this page on anti-particles if you are interested.] Let me quickly review the structure of matter, pulling it apart until we get down to the right levels.
- Atoms, about a billion times smaller in radius than your head, are made from electrons and atomic nuclei.
- Atoms can absorb and emit particles of light, called photons. This occurs through the electromagnetic force, for which the photon is the carrier (which means that photons are always in action when the electromagnetic force is operating).
- Atomic nuclei are made from protons and neutrons, 100,000 times smaller in radius than an atom, and made from mostly up and down quarks (and anti-quarks) and gluons.
- The protons and neutrons are kept intact, and also kept within an atomic nucleus, by the strong nuclear force, carried by the 8 types of gluons.
- The sun shines, and some atomic nuclei decay, because of processes that convert quarks of one type to quarks of another type, while emitting electrons and neutrinos, particles that stream straight out of the center of the sun.
- These quark-conversion and neutrino-emitting processes are caused by the weak nuclear force, whose carriers are the W+, W– and Z0 particles.
- The last force we know about is gravity, carried presumably by the graviton. Because of gravity’s astonishing weakness, this is not an easy particle to discover.
Almost every aspect of our daily world is determined by these particles. But there are a few more. The electron, the neutrino-1, the up quark and the down quark are called a single “generation” of particles — generation being used here loosely in the sense in which it applies to a family tree [though the particles do not carry any familial relationship that the word might imply.] There are two heavier generations, each carrying a heavier copy of these four types of particles.
- The second generation consists of the muon, the neutrino-2, the charm quark and the strange quark.
- The third generation consists of the tau, the neutrino-3, the top quark and the bottom quark.
The generational structure divides these particles into horizontal strata. One can also divide them vertically into those classes I mentioned: people often speak of “the electron-type particles” or the “charged leptons” to refer to the electron, muon and tau, speak of “the neutrinos” in general, and divide the quarks into the “up-type quarks” (up, charm, top) and the “down-type quarks” (down, strange, bottom).
You may wonder why the neutrinos have boring names compared to the rest of the particles. We used to call them something else, but we learned a lot of new things about neutrinos in the past 15 years, and we’re still in the middle of that learning process. Maybe when things settle down we’ll get them new names.
A type of Higgs particle was discovered in 2012, and by now we are quite confident it closely resembles the one predicted by the Standard Model. You can read some non-technical things about it in the Higgs FAQ 2.0, learn more details about its discovery here, and delve deeper into the theory through these webpages and these.
Let’s take a closer look at the various masses. Not only is the range of masses huge, there isn’t an easily discernible pattern. Here are some comments about the particle masses, starting with the lightest ones:
- The photon and graviton are probably massless — they must be astonishingly lightweight in order to allow the observed intergalactic magnetic field and the immense structures found in the universe.
- The gluon is as massless as you can meaningfully define it to be — gluons spend their lives trapped inside hadrons such as protons, and you can’t easily measure how light they are.
- Theorists long debated whether neutrinos were massless or not. Experiments of the last decade or so nearly settle the issue (though the evidence is still indirect so small loopholes remain). Neutrino masses are very tiny, with the heaviest at least a billion times lighter than the lightest atom (hydrogen), and the lightest one possibly much lighter indeed.
- The masses of the other elementary particles are known. The electron is roughly 1800 times lighter than hydrogen, while the top quark has a mass almost 400,000 times heavier than the electron, only a few percent less than a single atom of gold. And the W particle and Z particles are about half the mass of the top quark.
- All of the known elementary particles that have substantial masses get them directly from their interaction with the Higgs field. (The neutrinos may get their masses in a more indirect way, but the Higgs field is essential for their masses too.) I have indicated this with green borders of varying thickness on the particle disks.
We do not know the mass of the Higgs particle (or particles). The Large Hadron Collider should give us insights (and there are already hints).The Higgs particle’s mass was measured following its discovery in 2012.
I have sketched out the particles and forces in a different way in Fig. 2.
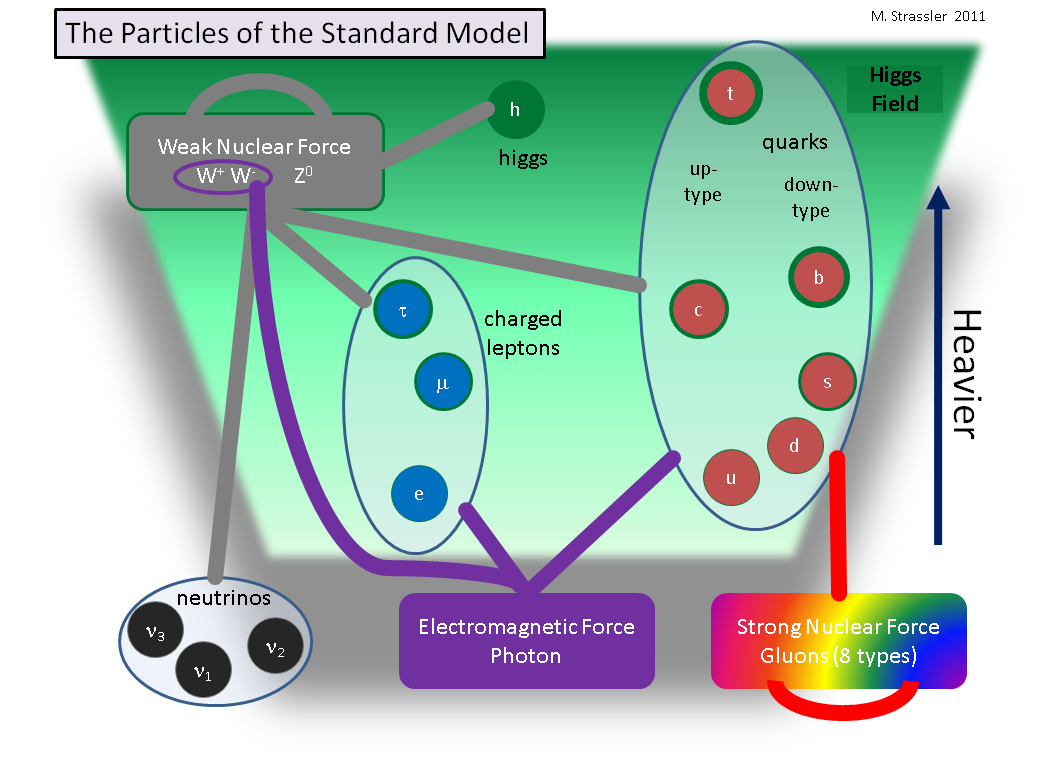
This figure shows which particles directly affect one another. In the figure I’ve drawn lines between all the types of particles that interact directly with one another. Here’s something interesting. Notice:
- None of what are often called the matter particles — charged leptons, neutrinos, or quarks — directly interact with one another.
- The matter particles only interact directly with the force carrier particles!
And this explains why the force carrier particles are so-named. When an electron in an atom interacts with an up quark inside the atomic nucleus, it does so indirectly. The electron interacts directly with photons, the quark interacts directly with photons, and the net (and rather complicated and initially counter-intuitive) indirect result is that the electron is pulled toward the quark and vice versa. Similarly, the force between two quarks is indirect, and stems from the direct interaction of quarks with gluons. All known forces between matter particles are indirect, and involve the mediation of force particles. When you push a door open, photons are at work.
The figure also captures a few other important features of the forces and the particle classes.
- All the particles of a given class are affected by the same forces; this is what defines them as a class, in fact. Neutrinos feel only the weak nuclear force; only quarks and gluons feel the strong nuclear force.
- Curved lines indicate that some of the force carriers interact directly with themselves or other force carriers. Note gluons interact with themselves, but the photon does not interact with itself (at least, not directly).
- There is a sense in which the Higgs particle is a force carrier too. But it’s a very special case. The stronger is the effect of the Higgs force on a particle, the larger is that particle’s mass when the Higgs field is not zero. (Note: The previous sentence is true for the known particles, but it may well be false for other as-yet-undiscovered particles.) I’ve indicated this tendency by showing the green sea as darker at the top of the page, indicating that it has a bigger effect on the heavier known particles. Similarly, the Higgs particle interacts with the heavyweight particles more strongly than with the lightweight ones.
This is an awfully strange-looking world, but like it or leave it: it’s ours. Although you can see some rough patterns, it is not exactly crisply organized. A lot of the disorganization turns out, in one way or another, to be associated with the Higgs field (or fields). You can read more about this association, and about what the world would be like if the Higgs field were on average zero, in this article.
8/8/11 Revised 6/3/22
134 Responses
LEAVING OUT one of the W-bosons (one particle is an anti-particle of the other, and no other anti-particles are mentioned too, so why make an exception here?) AND ADDING the graviton gives you: 6 QUARKS, 6 LEPTONS and 6 BOSONS. Am I # 6.666 reacting on this? 🙂
¿Puedes explicarnos màs sobre esto?, ha sido Genial encontrar mas datos sobre este tema.
Saludos
Hi there colleagues, how is the whole thing, and what you wish for to say about this paragraph,
in my view its really amazing in support of me.
Thank you for this espetacular job, Dr.Strassler!!
Thank you for a very clear explanation of these elementary particles.,
Ok now knowing all of this, what is possible? Knowing what something is made of is great, but using it I think is the interesting science we never hear about. Knowing each particle and their pros and cons what should be possible? Anti gravity? Free energy device perhaps? Assuming we can manipulate them there must be something exciting we can do with them.
hello,
just to say I liked this passage very much:
“the particles we know about and how they can be organized usefully into a few classes… a sort of periodic table of the particles, with a few twists”.
I would add “at a lower layer”, since as an IT engineer I’m so used with “layers” to see them everywhere, and then ask: how many more layers to go down thru?
regards,
corrado.
Thank-you for this article. I really appreciate your explanation on why “Force Carrier Particles” were so named. I was struggling to find something clear and concise. Thanks.
Hi, I recently read an article in “Scientific American” principally on gluons. The three researchers said that the oft repeated statement that the Higgs mechanism is the origin of mass in the visible universe is incorrect. They said further that the mass of quarks accounts for only 2 % of the mass of protons and nenutrons and that the rest apparently comes from gluons. My physics doesn’t go beyond an upper level undergraduate course in modern physics, but if the remainder of the mass comes from gluons, why isn’t that stated more clearly in the discussion of the Higgs mechanism? Is there some connection between the mass due to the Higgs mechanism and the mass due to gluons such that the Higgs mechanism engenders the mass caused by gluons? Thanks so much. You have an immensely interesting site here.
How about this paper:
http://arxiv.org/abs/1503.03290v1
Thoughts?
Sir, this presentation is great, illuminating and, of course intriguing. I thank you, Sir, very much for the writing.
Hi,Matt,,, I just want to know ,, you said earlier that quarks can convert from one type to another. Would it mean that quarks can further be divided?
i just wanted to know about the particle carrier of sound waves…do sound travelswith a particle..if yes then which particle?………As We all know Photon is particle carrier of light wave..so which is the particle carrier of sound wave
They’re called “phonons”. See http://en.wikipedia.org/wiki/Phonon
Arm yourself with a weapon designed for advanced combat and technology for hours of clandestine missions
– the Name of Obligation Black Ops II Gaming Mouse.
I’m curious tto find out what blog platform you’re utilizing?
I’m havng some minor secirity issues with my latest blog
and I would like too find something more secure. Do you have any recommendations?
Awesome issues here. I am very satisfied to peer your post.
Thank you a lot and I’m having a look forward to contact you.
Will you kindly drop me a e-mail?
What’s up, all is going well here and ofcourse every one is sharing data, that’s genuinely fine,
keep up writing.
Tremendous things here. I am very happy to see your
article. Thahk you a lot and I’m having a look forward to touch you.
Will you kindly drop me a mail?
It’s amazing designed for me to have a web site,
which is beneficial in favor of my know-how. thanks admin
Hey! I know this is kinda off topic however I’d figured
I’d ask. Would you be interested in exchanging links or maybe guest writing a blog
post or vice-versa? My blog goes over a lot of the same subjects as yours and I feel we could greatly
benefit from each other. If you’re interested feel free to shoot me an email.
I look forward to hearing from you! Awesome blog by the way!
Matt, something that has always puzzled me: how do we know the mass associated with a quark? What I am getting at is that the quark is always bound, and hence there is always binding energy, so what we measure is the sum of the energies of all quarks in a particle and the sum of all binding energies in the particle. Is the separation really just what we need to make the equations of the standard model work? Do we even know that the quark is a discrete particle, as opposed to, say, some sort of energy wave? There are a range of particles with a range of masses that superficially make little sense, although on the other hand there are equations out there that appear to predict these masses reasonably and they are not part of the standard model. (I put that there because just because an equation gets certain agreement with observation, that does not necessarily make it a correct assessment of nature.) Sorry if this is complicated.
At this moment I am going to do my breakfast, once having my breakfast
coming again to read more news.
Hi, constantly i used to check blog posts here in the early hours in the break of day, for the reason that i love to gain knowledge of more and more.
I think the admin of this website is genuinely working hard in favor of his website, since here
every material is quality based data.
Nice post. I learn something new and challenging on sites I stumbleupon every day.
It’s always helpful to read through articles from other authors and practice
something from their websites.
Matt, thanks very much for this information. I enjoyed reading it. As an interested person in quantum physics but with only a high school diploma, I struggle to make sense of the subject matter but enjoy the information all the same. I will continue looking for your publications and thanks for putting it on the net.
Hello matt
I red the whole article but I didnt get any thing about tachyons. Which I was hoping so could be here in ur article.
could u tell me please wats that ???
Tachyons are not in the Standard Model. So far as we know, there’s no such thing.
An impressive share! I’ve just forwarded this onto a coworker who has been doing a little homework on this.
And he in fact ordered me lunch due to the fact that I discovered it
for him… lol. So let me reword this…. Thank YOU for the meal!!
But yeah, thanks for spending the time to talk about this subject here on your blog.
why is everyone adamant about determining the nature of light; wavy or particulate? Why don’t we invert the idea; something like ,fundamental particles are made up of light.
This could be a good question , but I think light is the stream of photons, so what does it mean? Does it mean everything is made up of photons? Then how is it possible to find the bound state of photons? I think it would be impossible , because what would be the mediators for photons, which are also force carriers.
Share if you have more questions or suggestions.
Matt: You wrote “Gravitons do interact with everything”… That caught my attention. I thought that the graviton has not yet been “found,” and that we “know” nothing about its properties (other than a load of conjectures (fancy word for guesses)): When was the graviton detected, and by whom? Where can I read more about this?
The graviton is still hypothetical. However, if it exists, then by definition it interacts with everything. A particle that does not interact with everything would not be called a graviton.
Indeed; the graviton is to gravitational waves and gravitational forces as the photon is to electromagnetic waves and electric forces. Now, by the very nature of electrical forces, we know automatically that electromagnetic waves and the photons from which they are made interact with anything that has electric charge. Similarly, by the very nature of gravitational forces (Einstein’s version thereof) we know that gravitational waves (which we have indirectly observed, but have still not directly observed) and the gravitons that we *presume* they are made from [since all waves in a quantum world are made from quanta] will interact with anything that has energy and momentum. This universality of gravity at the level of forces is automatically transferred to the gravitons — if the gravitons exist. If they don’t exist, then there is something very fundamental about quantum theory that is wrong, and no one has ever made a consistent suggestion as to how our understanding of it could be *that* messed up.
Thanks for your help. I will now be able to sleep a bit better. I regret that I had not looked for laboratory confirmation of the doppler effect in light. I was hung up on how the doppler effect could be applied to particles. I had tried to get an answer from my neice who is a professor in astronomy but just got a pat on the head.
Thanks again
Roy
I am glad you mentioned quantum mechanics. That teaches us that light is a particle associated with a wavelength and not a wave. The doppler effect is concerned with waves originating in a medium where the source is moving relative to that medium and in my view it is difficult to marry that with photons. Photons of light are emmited from a body at the speed of light with an associated frequency, assuming no change they will reach an observer and will still have the same frequency. If there is a red shift what is the conclusion, either they are travelling below the speed of light or they have lost energy. Conversly a blue shift implies a gain in energy or they are travelling faster than the speed of light. So I have a problem whichever way I try to look at the origins of red shift. I should say that this line of enquiry started with our group wanting to understand Dark Energy and I started at looking at the basic assumptions of cosmology.
You can say what you want, but you should try to make contact with data. Gravitational redshift (which is what you are disputing) has been observed.
Both the Doppler effect and gravitational redshift can be observed in the lab, so there’s really very little room for doubt about the physics involved.
QM makes particles behave like waves and also makes waves behave like particles, so intuition based on the macroscopic world is typically of little use, but in this case there’s a fairly simple explanation: time dilation. Because a moving object measures time differently than a stationary one, it also measures frequency differently. The frequency of the photon as measured by the moving object is different than the frequency of the same photon as measured by the stationary one, and that’s the Doppler effect.
Similarly, General Relativity tells us that gravitational fields also affect the measurement of time. An observer on the ground measures time differently than an observer in orbit does, so (again) they measure the frequency of the same photon differently. And that’s gravitational redshift.
I understand the conventional wisdom and in the short term I have no difficulty accepting it. My problem is the assumption that no energy is lost to a photon over the vast distances of space. Is there no interaction with all the gravitational fields and background radiations out there that would result in some loss of energy in millions of years? Just imagine if ‘red shift’ was composed of two parts one due to doppler and one due to distance travelled, it would change cosmology, we would not need a ‘big bang’ we would have a steady state universe with stars being created and dying.
Quantum mechanics prohibits interactions of the sort you’re describing.
I am not a physicist but I run a Science and Technology group. I would like to pose a question which is bothering me. How does a photon manage to be so stable, with no loss of energy, over millions of years when it is travelling through space that is not entirely empty? If there was some loss or energy then would that be manifested as a reduction in frequency? If then a photon travelling through space produces a ‘red shift’ it would no longer be necessary for red shift to imply movement away from the observer which would change our view of cosmology.
No, individual photons don’t lose energy (and hence frequency) when traveling through not-quite-empty space. You can think of it this way: a particular photon either hits something en route to us or it doesn’t. If it does, it is scattered or absorbed and we never see it. If it doesn’t, it isn’t affected. (By way of analogy, a baseball doesn’t slow down if the batter swings but misses.)
Hi your website url: http://profmattstrassler.com/articles-and-posts/particle-physics-basics/the-known-apparently-elementary-particles/ appears to be
redirecting to a completely different webpage when I click the home page button.
You may want to have this looked at.
.grazie g………….ontosofiax memorialx eventux memorialx proust-heidegger…p
These particles are the low-energy effective particles, right? Do the “real” elementary particles in the standard model, i.e., before electroweak mixing, have names?
http://profmattstrassler.com/articles-and-posts/particle-physics-basics/the-known-apparently-elementary-particles/the-known-particles-if-the-higgs-field-were-zero/
I take it there’s no electroweak mixing without the Higgs? 🙂
Your question isn’t clear.
Sorry; carelessly stated, and besides which, if I’d read the article first the answer would have been obvious.
🙂 Yes, but I could also be reading a pattern where nobody has considered, because it sounds too absurd. Or any pattern looking for some application…
[code]=============== the (almost) complete pattern ====
level | fermions | u-type bosons | d-type | e-type | n-type
174.1 |n1, top | | | |
3.64 |n2, bottom | bb bs bd + anti | bu bc + anti | B- Bc- B+ Bc+ | eta_b B0 /B0 Bs /Bs
1.698 |tau, charm | | sc dc + anti | D- Ds- D+ Ds+ | eta_c D0 /D0
0.1219|mu, strange | ss sd dd + anti | su du + anti | K- pi- K+ pi+ | pi0, eta_8, K0 /K0
0 |e, up |
? |n3, down |
[/code]
Have you accounted for the fact that quark masses are renormalized as a function of distance scale? This is something that non-experts often leave out.
Ah, I was not worried about it here in the pattern, because it includes QCD quantities, so either it is something to be exploited at low energy, or it can not be exploited at all. The basic point in the table (by the way, good that the comments do support CODE and TEX tags!) is that the list of bosons is exhaustive and it matches the number of degrees of freedom of the fermion side, which is also exhaustive, so it could be used in some QCD superstring stunt trick or similar. To my regret, all the susy breaking stuff has been developed for the practical case of very massive scalar partners, so the literature does not have mechanisms with such mild breaking, nor even as exersice 🙁
A thing that people usually leaves out is that for equations involving mass quotients the running mass correction can be small, sometimes smaller that other second order effects equally unaccounted. For instance, in the above table I have choosen levels so that 3-4-5 and 5-6-7 fit Koide equation, and 4-5-6 is Koide using -sqrt(s) instead. Checking against http://prd.aps.org/abstract/PRD/v77/i11/e113016 , one can see that the difference between evaluating this last equation for [tex]\overline MS[/tex] [tex]m_q(m_q)[/tex] masses and masses al electroweak scale [tex]M_Z[[tex] is only a two percent; and the difference between the electroweak scale and the same quotient at GUT is only a 0.2%.
Looking at the diagram, mass ordered, sometimes I wonder if the neutrinos should really be at the levels of top, bottom, and down quarks… given that the other three leptons are near of charm, strange and up.
Data says what it says; neutrinos are lightweight. There’s no evidence to relate the neutrinos in any way with those three quarks. Also there’s no known reason why charm, strange and up should be combined into a family of three. You may be reading a pattern where none exists… as we humans are wont to do! 🙂
Thanks!
I really appreciate your feedback and insight:)
Thanks for you insight.
But I have a question.
Since this problem is not yet solved, do you think that I should continue researching? Would it be a waste of time?
Marie —
The question of why there is more ordinary matter than ordinary anti-matter in the universe (or at least in the part that we can observe — it may be much larger than that) is indeed an open problem, and you are welcome to work on it. However, you must also realize that hundreds of very smart people have tried already, and there are a number of quite reasonable suggestions that have already been made, one of which may be correct. So more precisely, it is not known to have been solved, but it **might** have been solved already. It’s not as though no one has any ideas.
Your original question asked “could the universe have its own anti-universe.” I presume your idea here is that there could be a second parallel universe with more anti-matter than matter, so that the two universes added together have equal amounts of matter and anti-matter. Well, there have certainly be suggestions that are similar to this. See for example: http://arxiv.org/abs/hep-ph/9904221 ; although this will be far too technical for you to read, just look at the last two sentences in the abstract describing this paper, and you’ll see the idea here is that there are two “branes” (like membranes, but with three spatial dimensions) that are literally parallel — like parallel lines — and are separated by a small distance in a fourth spatial dimension that we don’t yet know about. It’s almost (not quite) like two independent universes that are literally parallel. (The reason they are not independent is that objects on one brane attract objects on the other brane via ordinary gravity.) And the excess of matter (or “baryon number”) on the brane we inhabit is cancelled by the deficit on the other brane.
So I’m afraid that smart people have been here ahead of you… it is *really* hard to have a truly original idea, especially when you are just starting out. But you should not be discouraged. What you need to do is learn enough about the technical side of the science to be able to make proposals which go beyond words and ideas, and are grounded in the mathematics that we use to describe quantum theory and general relativity, and even more complex theories that have been proposed over time. That is probably (for the moment) the best use of your time, rather than actually trying to solve this problem now before you can really read technical papers about what other people have already tried. A few years from now, once you have that math and physics background, you will be able to apply it to all of these great problems, and a young mind that has few preconceptions is often the best one for making breakthroughs. So — good luck!
Marie,
first, as I see it, parallel universe (or extra dimensions hypotheses in general) has nothing to do with antimatter, at least directly. Antimatter can, and does, exist in observable universe, it doesn’t need a separate one. For instance, the very common process of neutron beta-minus-decay creates, among its other products, a particle called electron antineutrino.
second, you’re totally correct about the antihydrogen atom consisting of 1 antiproton and 1 positron.
third, and this is the most interesting part, is how it came to be that there is much more matter than antimatter in observable universe. I just checked Wikipedia (http://en.wikipedia.org/wiki/Baryogenesis) where it says that this problem is not yet solved. That is, the current Standard Model has no facility to explain this phenomenon.
Hi Matt, im 14… But im interested in partical and nuclear physics.
I have a questions regarding matter and anti matter. It is said that when a particle meets its anti partical they annihilate each other, transforming into energy. However, when our universe was created, matter somehwhat ‘overtook’ antimatter. For example a proton+ a electron= hydrogen atom, so does that mean that an anti proton+ a positron= anti hydrogen atom? what I am trying to say is that if a hydrogen atom can have its anti particle, just like how matter has antimatter, does that mean that our universe may also have its own ‘anti-particle’? In other words a parallel universe?
Thanks!
When you push a door open, (virtual photons are at work=disturbances in the electromagnetic field), do atomically bound electrons and nucleus bound quarks feel force? Does this (pushing a door open) change the kinetic and potential (interaction) energy of these electrons and quarks?
Hi Matt,
Great description – I’m 17 and trying to understand some of this… when I was alright until you said “When you push a door open, photons are at work”. So why exactly don’t we see ‘light’ randomly emanating from doors across the country?
Cheers,
Luke.
Oh! Good catch. I shouldn’t have said that in quite that way.
I should have said: virtual photons (disturbances in the electric and magnetic fields) are at work. I’ve spent all this time elsewhere on the website emphasizing that virtual photons aren’t really particles. http://profmattstrassler.com/articles-and-posts/particle-physics-basics/virtual-particles-what-are-they/
So you caught an inconsistency in my explanations; a consequence of the order in which the articles were written. Thanks, I’ll fix that.
As far as I can see the mysterious parameters of the particle’s rest masses are now replaced by the corresponding mysterious parameters of their interaction strength with the Higgs field. Is there some way in which the Higgs field is making anything more clear and less arbitrary?
Your question is on target, and your impression is absolutely right. The Higgs field is necessary so that the masses of W and Z particles, quarks and leptons can be non-zero, but (with the exception of the W and Z particles themselves, whose masses are related to a combination of the value of the Higgs field and the strength of the electromagnetic and weak nuclear forces) it provides no explanation for the actual values of the masses of the quarks and leptons… no reason why the top quark should be 400,000 times heavier than the electron. That is one of the key problems in particle physics today, for which perhaps the LHC will give us some guidance.
But another point which is a little harder to explain is that the Higgs field doesn’t just give masses to things — it rearranges the forces. (See http://profmattstrassler.com/articles-and-posts/particle-physics-basics/the-known-particles-if-the-higgs-field-were-zero/ ) And it does so in a very precise way, one that has been carefully verified over the years, especially at the SLC and LEP colliders (at Stanford and CERN, respectively.)
So don’t get the impression that the only thing the Higgs field is good for is giving masses to a bunch of particles. There’s much more going on.
In Cosmic Variance article that links here you actually mention the “equations of standard model” but this article is about the particles not about the equations. I am trying to understand how much of what is done in LHC is done by computer programming and modeling and how much of it is actually “standard model equations.”
Well — that’s a very complicated story. I don’t think I could put up a page about the equations of the Standard Model on a public website — learning how to use the equations of quantum field theory was the single most complicated intellectual leap that I ever made as a student. I am still learning new things every time I try to teach a course on it. We’re talking about taking limits in applied infinite dimensional calculus… and other nasty things…
At LHC: there is some modeling of the proton that has to be done, but most everything else is an attempt to carry out bona fide equations, not modeling. That said, the calculations are very complicated, often involving integrals over many variables, and they can’t be performed analytically. And certain approximation schemes are always being applied. But the calculations are all based on analytic equations (except for the structure of the proton, which has to be modeled using past experiments.)
well thanks for your answer! To understand that there are two different particle-wave duality problems is a great start…I liked the bit about notions that are unfamiliar…but appropriate for nature! This is the very thing that makes it hard for folks like me to follow (and for you to explain), but without that I somehow doubt I would be that interested in knowing (if it’s familiar it risks being boring)
Just to be one step clearer — there aren’t two particle-wave *duality* problems, but rather two different precise contexts in which both waves and particles appear. There are equations to go by. Wave-particle duality, by contrast, is a conceptual approach to thinking about nature and the corresponding equations, not itself backed by any specific equations, arguing that to understand nature properly you have to understand that an electron has wave-like properties as well as particle-like properties, and the same is true for photons, Higgs particles, etc.
I could probably say that better too. Sigh…
And I thought I was beginning to understand… Which view is “right” as far as understanding the fundamental nature of the universe, quantum mechanichs or quantum field theory? I can see that both can be “right” simoultaneously as far as their “equations to go by” make accurate predictions in their respective areas of application, but that’s not fully satisfactory… Let me rephrase the question. When we talk about the elementary particles, the elementary constituents of the world, including the Higgs bosson, are we mainly talking about those “quanta” of QFT? Or is there an alternative view based on quantum mechanics? And if there is, are both interchangeable? Or is one of the theories subsumed by the other? It seems like there is no wave-particle duality “problem” in quantum field theory, just “quantized waves/fields”, with no need for particles, except maybe as a metaphor. But then how should one interpret things like the quantum double slit experiments, the idea that measuring some magnitude “collapses” a probability (the interpretation of the wave function in quantum mechanics) into a “real” value for that magnitude, the uncertainty principle, the “superposition” of states in e.g. quantum computers, etc. i.e things that suggest that there is such duality.
I’m probably mixing things up badly, sorry about that. I thought I understood the wave-particle duality as explained in e.g. http://physics.about.com/od/lightoptics/a/waveparticle.htm, but not any longer after reading your (very clear) description of the differences between quantum mechanics and quantum field theory.
Hmm…
There’s a risk of semantic confusion, so let’s first eliminate that.
The term “quantum mechanics” has two uses. One is as the general category of quantum techniques, of which quantum field theory is one application. String theory would be another application. The second use is in its specific application to discrete objects that we think of as point-particles; this is what we learn first in school, for example for describing electrons in atomic physics. Let’s call that “1920s quantum mechanics” to avoid confusion.
In other words, 1920s-quantum-mechanics and quantum-field-theory are both examples of the general class of things that operate according to the general principles of quantum mechanics.
Now: 1920s quantum mechanics is inconsistent with relativity, both special and general. The only way to bring them special relativity into quantum mechanics is to use quantum field theory. So yes, 1920s quantum mechanics has to be supplanted by something more general. Doing so has many benefits, including explaining many puzzles of 1920s quantum mechanics, predicting the positron [the electron’s anti-particle], and allowing (for example) for a full quantum theory of both light and electrons, which isn’t possible in 1920s quantum mechanics. And many aspects of the world cannot be calculated successfully, or at all, using 1920s quantum mechanics, while quantum field theory does very well.
Indeed, 1920s quantum mechanics was obsolete by the mid-to-late 1930s. However, I should note that it wasn’t clear that quantum field theory was entirely the right framework for all of the non-gravitational forces until the 1970s; indeed most people in the 1960s didn’t think it was.
The question of how the physics of particles in quantum field theory reduces down, say in atomic physics, to what you know about the behavior of particles in 1920s quantum mechanics is not a trivial one at all. (It’s also not trivial to see how Newton’s law of gravity emerges from general relativity — it takes some real work.) I don’t think I can give you a non-technical explanation of this. For instance, in 1920s quantum mechanics one speaks of position and momentum for particles; in quantum field theory one does not, and the language of 1920s quantum mechanics therefore has to emerge in a complicated way. And in quantum field theory the wave function is not a function of particle positions but of the field values across all of space… it’s such a messy object that no one ever uses it.
I think the best thing for me to say right now is that I don’t feel that I myself can explain this clearly enough to answer your question right now. Thinking of a good way to do so is on my list of things for the future. A technical discussion of bound states (i.e. something like an atom) appears in some quantum field theory books, such as Peskin and Schroeder… but I assume you want a non-technical discussion.
Hi Matt, thanks for the great website. I stumbled upon your guest-post on Cosmic Variance and have been fascinated by your posts ever since.
I have a question that may sound extremely silly (then again, I’m a molecular biologist and way out of my field around here). The way I understood it from your posts, the set of equations that form the “Standard Model” describe the physical world in particles, by “particle” meaning the smallest manifestation of a field allowed by quantum mechanics. How is the Standard Model unified with the so-called wave-particle dualism? Does this present a problem at all?
(I greatly suspect my problem with thinking clearly about this issue is that I’m overly influenced by the “common sense” meaning of the words particle and wave, both probably a misnomer for irreplaceable but overly complicated equations)
Welcome, Davide. You are right that common-sense notions make things complicated, but even worse than that, there are two different particle-wave things going on here. It makes things extremely confusing for everyone, even graduate students as they’re learning the business.
First let me answer the easy question: “How is the Standard Model unified with the so-called wave-particle dualism? Does this present a problem at all?” No problem at all; wave-particle dualism (which has to do with the wave-function of quantum mechanics) is incorporate into the very structure of quantum field theory (in which particles are viewed as quantized ripples, or “quanta”, as Einstein dubbed them when he first invented the concept of a photon.) Since the Standard Model is an example of a quantum field theory, no issue arises.
What is extremely messy is that quantum mechanics (which is what most people learn and/or read about) gives intuition about particles and waves and the relationship between them that is quite different from the intuition that one learns from quantum field theory. So all this reading about what Bohr and Einstein were saying back in the 20s and 30s has a big problem: in some ways, it is out of date, at least linguistically. I’m struggling with how to deal with this problem on the website; I think it is going to need a long article, but I’m not sure what it is going to say.
Here’s a (probably incomprehensible) preview:
The concept of particle in quantum mechanics, before you do quantum field theory, is quite a bit more like the Newtonian concept of a particle; and the wave that shows up in quantum mechanics is the wave function, which is not a function in ordinary space at all, but a function in the space of possibilities. (A wave function for one particle in quantum mechanics is a function of 3 dimensions, but a wave function for two particles is a function of 6 dimensions — because the space of possible positions of two particles is specified by x1,y1,z1,x2,y2,z2) Wave-particle duality arises from having a wave function evolve smoothly and describe probabilistically what a particle might be doing.
Whereas, in quantum field theory, there’s still a wave function (of an infinite dimensional space of possibilities now — eeeek!!) but that’s not the type of wave I’m talking about in these articles. Even in classical field theory, before quantum mechanics, there are waves — water waves, waves on a violin string, sound waves, electromagnetic waves — and these are waves in ordinary space, three-dimensional waves, ripples on a field. Now when we go to quantum field theory, quantum mechanics demands these ripples have a quantized height; they can have a minimal size, or twice that size, or three times that size, but not 2.74 times that size. Einstein called such things “quanta”. They’re really not what our intuition imagines for “particle”. But in many ways they do behave like “particles” — they have definite energy and momentum, they bounce off of things, they can be emitted or absorbed — and so we retain the word “particle” for them. Maybe it would have been better to stick with “quanta” and avoid linguistic confusion, but then again, particle intuition is often useful for them. Anyway, what’s going on here isn’t really the wave-particle relationship of quantum mechanics; it’s ordinary waves, not a wave function, and an unfamiliar (but appropriate for nature!) notion of particle, not the one taken for granted in one’s first quantum mechanics class.
And worst of all — the wave-particle duality concept that Bohr developed partially draws on both of these wave-particle relations! Ugh! Very hard to tease this apart. I guess someday I’ll have to do it very carefully; then I’ll try to explain it clearly to you.
If you were to add gravity and gravitons to this diagram, would they interact with everything? Even massless photons can be bent by gravity, right?
I’ve heard it said that the electromagnetic force is “long-ranged” because photons do not interact with themselves, whereas gluons and W/Zs do. Would it be right to generalize and say that gravitons must not interact with themselves either because gravity is also “long-ranged”?
So then… gravitons interact with everything except themselves?
Good guess but not so. Gravitons do interact with everything *including* themselves. In fact that’s a key reason why Einstein’s theory differs from Newton’s (though Einstein didn’t phrase it that way initially.)
It is not true that “the electromagnetic force is `long-ranged’ because photons do not interact with themselves, whereas gluons and W/Zs do.” I’m not sure who told you that; it’s simply false. The question of whether a force is long range is quite separate from whether the corresponding particles have self-interactions. You can have self-interacting particles that generate long-range forces; there’s a whole subject of study called “conformal field theory” which is all about that. Or you can have short-range forces from photon-like particles that do not self-interact; in fact, inside a superconductor a photon does generate a short-range force, through a Higgs-like phenomenon first understood by Anderson, a year or so before Higgs (and some other particle physicists too, who should not be forgotten) considered the question. (However, Anderson did not predict a Higgs-like particle as a consequence, I believe.)
Thanks Matt – after posting the above, I found your article on SN1987A. I realize that the small uncertainty in arrival time leaves a small possible mass. I have a few other questions, if you wouldn’t mind looking at them. The SN1987A neutrinos velocity discrepancy was about 10^-9 or 10^-12, I remember, depending if you looked at arrival time or energy width. In the paper by Cohn & Schalow, they remark that the existence of TEV neutrinos indicate that the Bremstrahlung effect limits their velocity discrepancy to about that of the SN1987A neutrinos. Very close to C at low energies and at high energies. Doesn’t Occam’s Razor suggest that the velocity is pretty much the same or identical for all energies?
The second question I had (maybe it is also addressed somewhere on your site) is the relation between tachyons and superluminal neutrinos. I guess I thought that all FTL particles were tachyons, but isn’t a tachyon supposed to go faster as it looses energy? Due to Bremstrahlung energy loss, all particles would accelerate and thus lose energy faster and etc. Maybe this is due to using the eqns of special relativity, and we’re not supposed to apply them to the OPERA neutrinos.
Hi Matt – This is a very interesting site. My training was in plasma physics, but I’ve developed an interest in particle physics since the announcement of (apparently) superluminal neutrinos from the OPERA experiment. My question has to do with your statement that neutrinos have mass. Hasn’t the results of SN1987A shown that to a very small uncertainty neutrinos (at least the ones observed in1987) travel exactly at the speed of light? And if so, doesn’t that mean they are massless?
No, the fact that supernova neutrinos travel very close to the speed of light to a very small uncertainty only means that neutrinos are massless to a very small uncertainty. And now we have to work out how big that very small uncertainty is.
The neutrinos from the supernova had energies around 10 MeV or so (that’s 10,000,000 eV). They arrived within a few seconds of one another. As scientists calculated almost immediately, that indeed put a limit on the supernova neutrino’s masses: they could not be larger than about 30 eV/c2. See http://adsabs.harvard.edu/abs/1988neph.work..306M
However, we have better limits from cosmology today: the limit is closer to 1 eV/c^2. And we have evidence from neutrino oscillations (http://profmattstrassler.com/articles-and-posts/particle-physics-basics/neutrinos/neutrino-types-and-neutrino-oscillations/) that neutrinos do have mass; at least one has mass around or above .1 eV/c^2.
So what is the lesson? In physics, you must be quantitative about what you do not know, if you are to come to correct quantitative conclusions about what you do know.
Prof. Strassler,
I see how the matter particles don’t ever interact with each other, and I can see that when you push open a door it must be photons working to mediate the interaction between your particles and the door’s, since it isn’t the strong or weak nuclear forces. Can you explain how exactly photons work to move the door, or how they carry the force from you to the door? Thanks.
Did you read my article on “virtual particles” yet? It’s not photons (the particles of the electromagnetic field) that do the job, but more general disturbances in the electromagnetic field (typically called “virtual photons” even though they are really not photons at all.) To explain why a disturbance can do this requires simple equations, but not zero equations. For instance, nothing I say in words can explain to you why the disturbance between two electrons leads to a repulsion, while the disturbance between an electron and a proton leads to an attraction.. To understand that you must do a calculation — words fail at precisely this point.