At a conference like LHCP12, covering all of Large Hadron Collider [LHC] physics and beyond, there’s far too much to summarize: hundreds of talks, with thousands of incremental experimental results and theoretical insights. So instead, today I’ll draw attention to one of the longest-running puzzles of the LHC era, and to a significant step that’s been made toward resolving it. The puzzle in question involves a rare decay of bottom quarks.
[All figures in this post are taken from LHCP12 talks by Zhangqier Wang and Eluned Smith.]
The Decay of a Bottom Quark to a Lepton-Antilepton Pair
In the Standard Model of particle physics, bottom quarks most often decay to charm quarks. They do so via a “virtual W boson” — a general disturbance in the W field — which subsequently is converted either
- to a quark-antiquark pair, or
- to a “lepton” (an electron, muon or tau) and an anti-neutrino.
[See for instance Figure 1 of this post.]
But rarely, a bottom quark can decay to a strange quark and to a lepton-antilepton pair (an electron and a positron, or a muon and an anti-muon, or a tau and an anti-tau.) The example of a muon-antimuon pair is shown below.
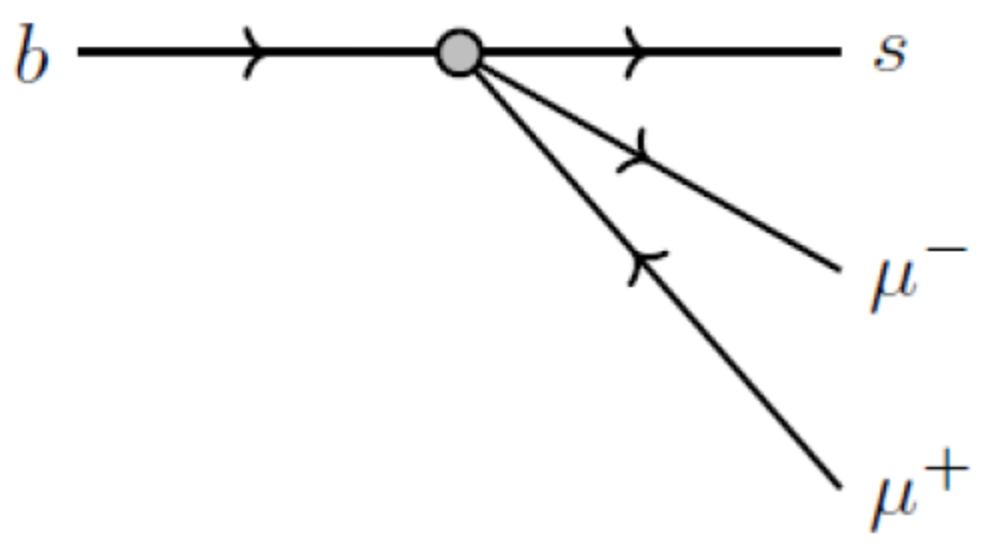
Within the Standard Model such a process can occur through quantum physics, involving subtle interactions of the known elementary fields. It is very rare; less than one in a million bottom quarks decays this way. But it can be measured in detail.
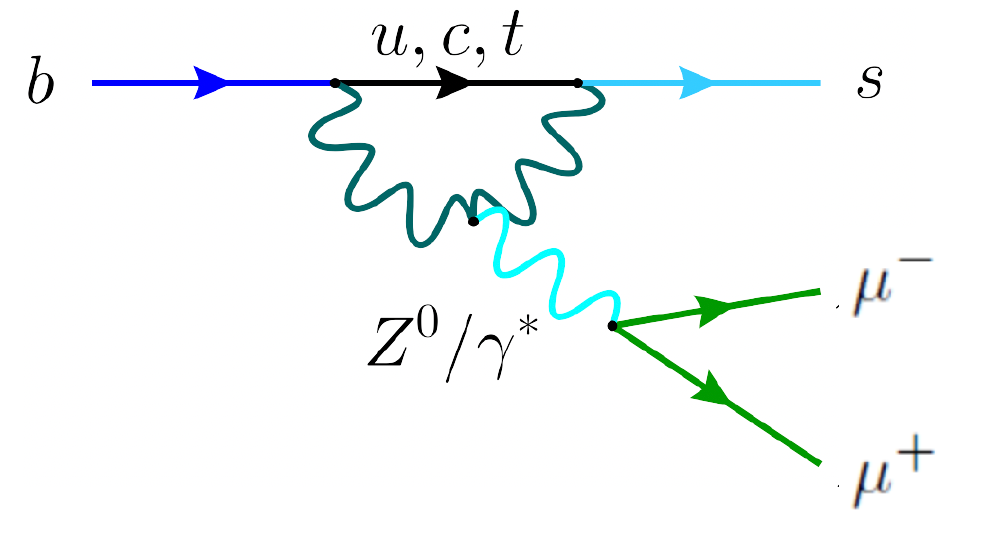
Because it is rare, the rate for this process is easily altered by new particles and fields that aren’t included in the Standard Model, making it an interesting target for theorists to explore. And since the process is relatively easy to measure, it has been a key target for experimentalists at the LHC experiments, expecially LHCb and CMS, and to some degree ATLAS.
The Discrepancy at the LHCb Experiment
For a decade, theorists’ predictions for this decay have been in conflict with the measurements made by the LHCb experiment. This is quantified in the plot below, which shows a certain aspect of the process as a function of the invariant mass-squared (q2) of the muon/anti-muon pair. (More precisely, as shown at the bottom of Figure 1 of this post from 2013, the measurement involves a B meson decaying to a K meson plus a muon/anti-muon pair)
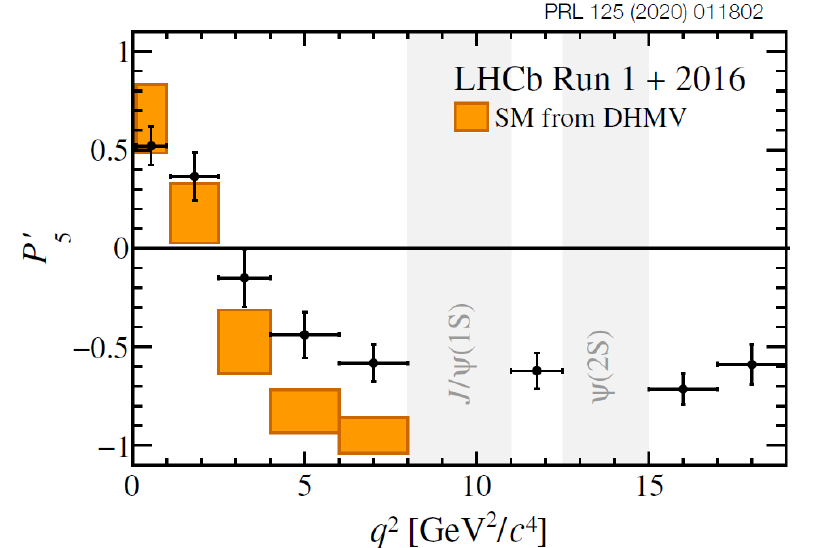
What are we to make of this disagreement? Well, as always in such situations, there are three possibilities:
- The experimental measurements have a mistake somewhere,
- The theoretical calculations behind the prediction have a mistake somewhere, or
- The Standard Model of particle physics is missing something
The third case would be of enormous importance in particle physics: a discovery of something fundamentally new, and a cause for celebration. The first two options would be far less exciting, and we must rule both of them out convincingly before celebrating.
If there is an error in the measurements or calculations, it is unlikely to be something simple. The people involved are experienced professionals, and their work has by now been checked by many other experts. Still, subtle mistakes — an underestimate of a complex quantum effect, or a feature of the experimental detector that hasn’t been properly modeled — do happen, and are more common than true discoveries.
The Contribution of the CMS Experiment
Importantly, we can now rule out the first possibility: there’s no mistake in the LHCb measurement. The CMS experiment has now repeated the measurement, with much improved precision compared to their previous efforts. As shown in Fig. 4, the LHCb and CMS measurements match. (CMS and LHCb are so different in their design that there’s no reasonable possibility that they have correlated detector issues.)
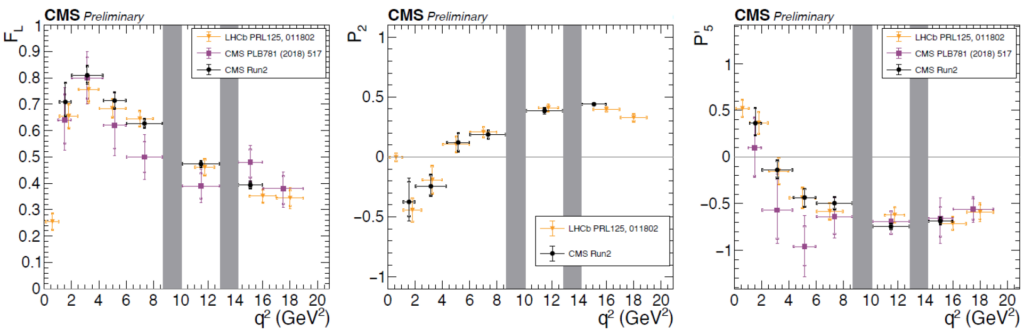
Since the experiments agree, focus now moves squarely to the theorists. Are their predictions correct? We have at least two sets of predictions; they appear as blue and orange bars in Fig. 5, which shows they agree with each other but disagree, in the center and right panels, with CMS data (and therefore, from Fig. 4, with LHCb data.)
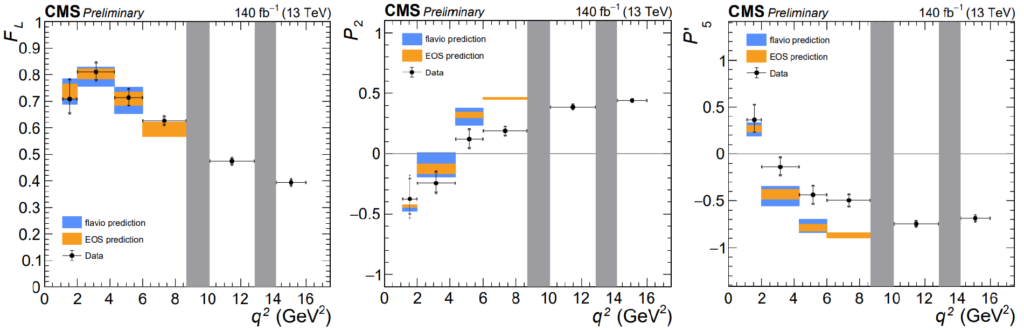
Even though the two theoretical calculations agree, they are based on similar assumptions. Perhaps those assumptions are flawed?
There are certainly things to worry about. Anything involving the strong nuclear force, when it acts at distances comparable to the size of a proton, has to be subjected to heavy scrutiny. Far too often, discrepancies between theory and experiment have dissolved when potential theoretical uncertainties from the strong nuclear force were reconsidered. (For a recent example, see this one.) We will have to let the theory experts hash this out… which could take some time. I would not plan to order champagne any time soon. Nevertheless, this bears watching over the next few years.
5 Responses
Bottom Quark Decay
[edited by host]
CORRECTION;
[edited by host: putting up half-baked, incomplete calculations, no matter what your personal theory of the universe, are not helpful to anyone. This is not what serious physicists do.]
One does not normally see isolated quarks.
Should we imagine the quarks in figure 2 as being in a sea of quarks and gluons – as per your diagam here https://profmattstrassler.com/wp-content/uploads/2013/04/nucleons31.png
Yes, the decay of the bottom quark always occurs within a hadron that contains a bottom quark and a bunch of light quarks, anti-quarks and gluons. This hadron would be called a “B meson” if it is a boson and a “B baryon” if it is a fermion (with three extra quarks, like a proton.)
Although much of these assertions are beyond my scope of computations, indeed understanding, as you say, where there is ‘worry’ or considerations that needs to be ‘scrutinized.
Unseen forces, as an example, although still knowing of their effects within and external to their environments, from an experimental basis, removing theory, still exhibits inconclusive results including discrepancies and uncertainties, unless the laws that govern such forces are understood. Coming from a theological experiment, removing theory, helps me understand things unseen as though they are seen because of the effects it has on the nature of my spirit which receives ‘forces’ external from myself to help me identify, similar to empirical models, discerning truth from error.
Dr Strassler’s quote: There are certainly things to worry about. Anything involving the strong nuclear force, when it acts at distances comparable to the size of a proton, has to be subjected to heavy scrutiny. Far too often, discrepancies between theory and experiment have dissolved when potential theoretical uncertainties from the strong nuclear force were reconsidered