Matt Strassler [March 4, 2013]
Now that we know a nucleus is tiny, we have to ask an obvious question: why is it so small? Atoms are made from tiny particles, but they are much larger than the particles they contain. We learned why in this article. By contrast, nuclei are not much different in size from the protons and neutrons that they are made of. Is there a reason, or is this a coincidence?
Meanwhile, we know already that electrical forces hold atoms together. What force or forces are holding a nucleus together?
Here we begin to enter new territory, far different from what we’ve explored previously… because it is clear that a new force that we have not yet discussed must be at work.
The Residual Strong Nuclear Force
If nature had only gravitational and electrical forces, the ones we encounter in daily life, a nucleus with multiple protons would blow itself apart: the electrical forces pushing the protons away from each other would be millions of millions of millions of times stronger than any gravitational forces pulling them together. So some other force must provide an attraction even stronger than the electrical repulsion. This force is the strong nuclear force — though only a shadow of its true power will be visible in the structure of the nucleus. When we study the structure of protons and neutrons themselves, then we will see the true capabilities of the strong nuclear force. In the nucleus, we encounter only what’s sometimes called a “residual force” — and I will call it the “residual strong nuclear force”. (Sometimes this term is not used — people just call it the strong nuclear force, but there’s merit in this distinction.)
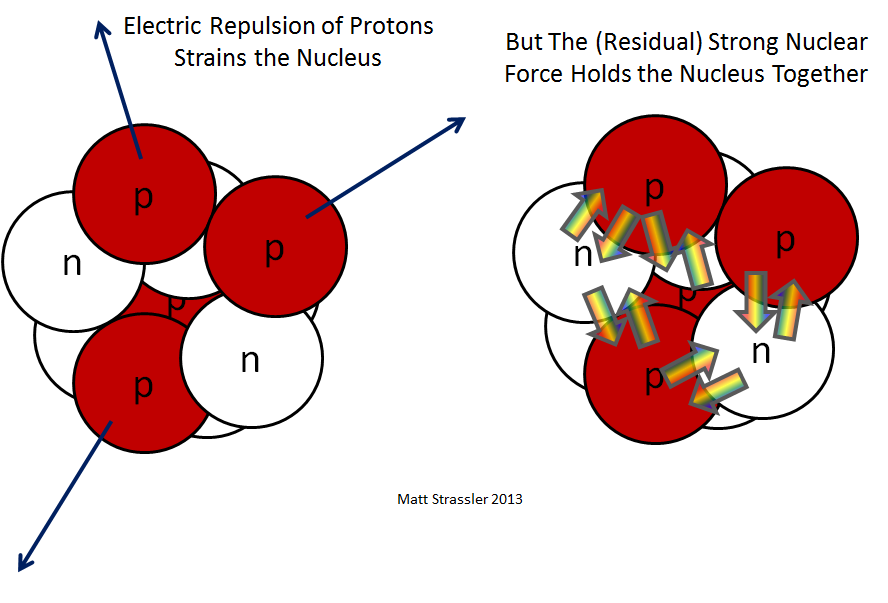
A caution: In the end, although (as we’ll see) the full-fledged strong nuclear force — the force between the particles (quarks, gluons, anti-quarks) inside a proton or a neutron — is fairly simple, at least in some senses, the residual strong nuclear force is a complicated residue of various cancelling effects, and consequently there is no simple picture that describes all of the physics of a nucleus. This isn’t surprising, when we recognize that protons and neutrons are internally complicated. There’s something of an analogy with atoms and molecules.
In an atom, tiny nuclei and even tinier electrons are relatively far apart compared to their sizes, and the electrical forces that hold them in the atom are simple. But in molecules, the distance between atoms is comparable to the sizes of atoms, so the internal complexity of the atoms comes into play. A diverse and complicated set of partially cancelling electrical forces, and processes in which electrons may actually move from one atom to another, make the story of molecules much richer and more complicated than that of atoms. In a similar way, the distance between protons and neutrons in a nucleus is comparable to their size — and so, as with molecules, the forces that hold nuclei together are much more complicated (in some senses!) than the forces that hold protons and neutrons together.
When we learn about the structure of protons and neutrons, this story will start to become somewhat (but only somewhat) clearer. Basic features of nuclear physics are well understood, but the subject remains very technical, and many of the details are still undergoing active research. I won’t be able to do it justice in this article, partly because I’m not expert enough to simplify it wisely for you… though perhaps one of my nuclear physicist colleagues can assist me.
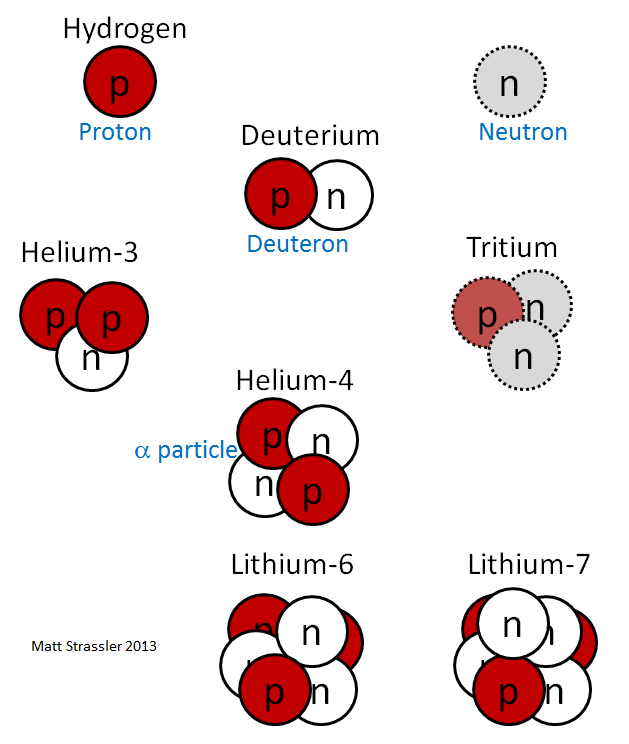
A Pattern
Let’s see what we can learn from simple considerations about how this force works. One clue is that all nuclei except that of the most common isotope of hydrogen (which has only one proton) contain neutrons; that is, there are no nuclei with multiple protons that do not contain neutrons. (See Figure 2.) So clearly the neutrons play an important role in helping the protons stick together.
Conversely, there are no nuclei made from only neutrons and no protons; most lightweight nuclei, like those of oxygen and silicon, have about the same numbers of neutrons and protons (Figure 2). Larger nuclei with larger masses, like those of gold and radium, have somewhat more neutrons than protons (Figure 3). This suggests two things:
- Not only are neutrons needed to make protons stick together, protons are needed to make the neutrons stick together too.
- If the number of protons and neutrons becomes very large, then the electrical repulsion pushing the protons apart has to be compensated by the addition of a few extra neutrons.
This last statement is illustrated in Figure 3 [taken from Wikipedia] which shows the stable (black) and relatively long-lived though unstable (colored) nuclei, as a function of the number of protons Z and the number of neutrons N that they contain. Notice the stable nuclei have Z and N approximately equal for small Z and N, but N becomes gradually larger than Z as the two increase. Note also that the band of stable and long-lived unstable nuclei remains quite narrow for all values of Z. Despite the immense progress in nuclear physics over the past 80 years, there is no widely-accepted simple explanation of this remarkable fact. I believe it is viewed by most experts as an odd accident.
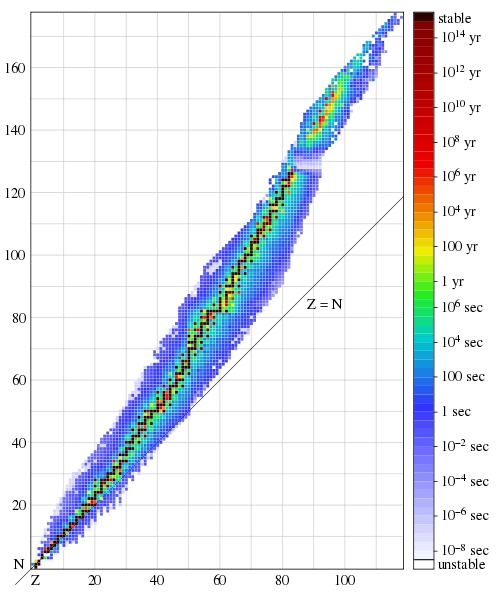
The Size of a Nucleus
One of the main purposes of this article was to explain why nuclei are small compared to atoms. [Later on we’ll learn why protons and neutrons are small.] To do this, let’s start with the simplest nucleus that has both protons and neutrons: this is the second-most common isotope of hydrogen, consisting of one electron (as for all hydrogen isotopes) and a nucleus made from one proton and one neutron. This isotope is often called “deuterium”, and the nucleus of deuterium (see Figure 2) is sometimes called the “deuteron.” How should we understand what holds the deuteron together? Well, we might naively imagine that it is not so different from a hydrogen atom, which also contains two particles (a proton and an electron). See Figure 4.
As we saw in an earlier article, the fact that electrons have a small mass compared to protons and neutrons assures that
- the mass of an atom is essentially the mass of its nucleus,
- the size of an atom (essentially the size of the electron cloud) is inversely proportional to the electron’s mass and inversely proportional to the overall strength of the electromagnetic force; the uncertainty principle of quantum mechanics plays a crucial role.
What about the deuteron? It is similarly made from two objects, but these are of almost equal mass (the neutron and proton’s mass differ by only one part in about 1500, for reasons we’ll learn later) so both are equally important in determining the deuteron’s mass and its size. Now suppose there were a new force pulling a proton toward a neutron that was much like the electromagnetic force (it isn’t quite like that, but imagine it for a moment); well then, by analogy with hydrogen, we’d expect the deuteron’s size to be inversely proportional to the mass of the proton or neutron, and inversely proportional to the strength of the new force. If the force were just as strong (at a particular distance) as electromagnetism, that would mean, since the proton is about 1850 times heavier than the electron, that a deuteron (and indeed any nucleus) should be at least a thousand times smaller than hydrogen.
But we’ve already guessed that the residual strong force is much stronger than electromagnetism (at the same distance) — because if it weren’t, it wouldn’t be able to prevent the electromagnetic repulsion among the protons from blowing larger nuclei apart. So that extra strength is going to pull the proton and neutron even more tightly together. And thus it’s not surprising that the deuteron and other nuclei are not just one thousand but tens of thousands of times smaller than atoms! Again, this is just because
- protons and neutrons are nearly 2000 times heavier than electrons
- at these distances, the strong nuclear force between the protons and neutrons of a nucleus is many times stronger than corresponding electromagnetic forces (including the electromagnetic repulsion between the protons in the nucleus.)
This naive guess gives roughly the right answer! But it doesn’t fully capture the complexity of the interaction between the proton and neutron in deuterium. One obvious problem with it is that a force similar to electromagnetism but with greater pulling or pushing power would have an obvious impact on daily life, and we don’t observe any such thing. So something about this force must be different from electrical forces.
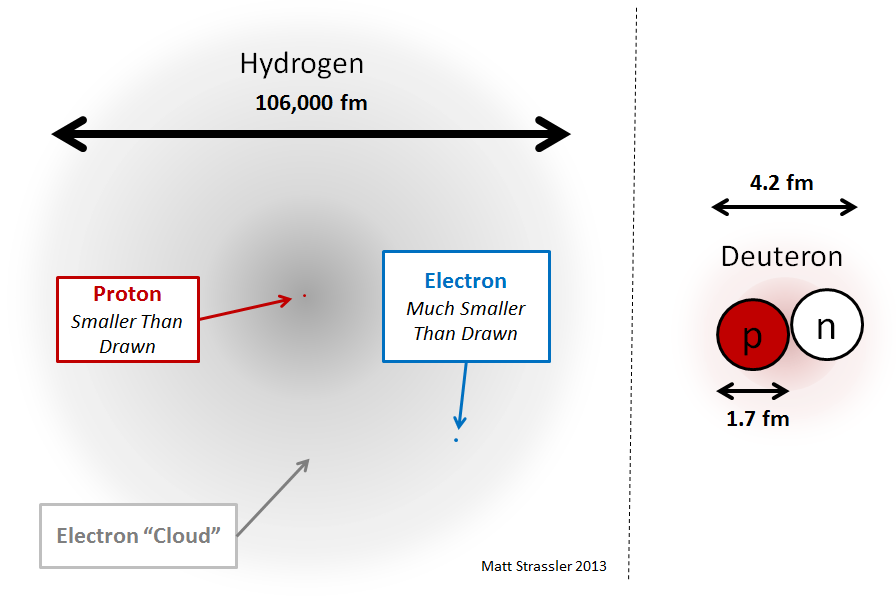
The Short Range of This Force
What’s different is that this residual strong nuclear force is very important and powerful for protons and neutrons that are a very short distance apart, but beyond a certain distance (called the “range” of the force) it falls away very rapidly, much more rapidly than electromagnetic forces do. The range — somewhat by coincidence — turns out also to be about the size of a moderately large nucleus, just a few times larger than a proton. If you bring a proton and a neutron together at a distance comparable to that range, they will attract each other and form a deuteron; if you leave them at greater distances, they will barely feel any attraction at all. (Actually, if you bring them too close together, so that they start to overlap, they will in fact repel each other; heck, I warned you the residual strong nuclear force was complicated!) In short,
- the residual strong nuclear force is much, much weaker than electromagnetism at distances significantly greater than the size of a typical nucleus, so we don’t encounter it in daily life; but
- at shorter distances comparable to a nucleus it becomes much stronger — an attractive force (as long as the distance is not too short) able to overcome the electric repulsion between protons.
Later on we’ll learn something about why this force is only important at distances comparable to the size of a nucleus.
Larger nuclei are held together by more or less the same force that holds a deuteron together, but the details are complicated, technical, and not easy to describe. Nor are they fully understood. Although the basic outlines of the physics of nuclei have been well understood for decades, many important details are still active subjects of research.
At some point, if and when I learn more things that may be intuitively easy to understand, I may add more to this article. But for now it is time to move on — to a description of protons and neutrons, where the strong nuclear force reveals its true colors. [Coming Soon]
39 Responses
As I understand, the ‘residual’ strong force mediated by pions is stronger than the EMrepulsive force at 1fm thus holding together the nucleus. How strong is the residual force compared to the color force confined to the inside of the nucleons. I have read that the strong force is 137 times stronger than the EM force but am not clear if that ratio refers to the color force inside the nucleon or the binding for e between nucleons. Also what is the physical explanation for the repulsionof the strong force at distances less than 1 fm?
In your article, it is noted that strong nuclear interaction is a very complex phe-nomenon. In particular, a graph is given of the dependence of the number of pro-tons on the number of neutrons in stable nuclei. You indicated that this graph has no convincing explanations. However, these explanations are easily given by the Unified Theory of Nature (see my-gravity.com). Its power and universality is proved on 140 examples of its application (see Universality of the Unified Theory). Part of the answers to the riddles in your article are outlined in Nuclear Interac-tion and Theoretical Physics, Nuclear Interaction and Unified Theory. A little later I will publish the missing answers.
The fact is that balance of forces between neutrons in nucleus is impossible.
For example He-4 nucleus is a rotating complex of four nucleons (attracted to each other by the strong nuclear force), of which neutron A and neutron B. A simple analysis of the attracion force and the centrifugal force indicates that balance is inpossible for any stereo metric complex. Considering tetrahedric symmetry neutron A is attracted by the other three nucleons by a consistent force, perpendicular to the rotation axe, so that the attracion can be balanced by a centrifugal force. However the location of neutron B has not possibility for balancing the attraction by a centrifugal force.
Is the QCD concept correct?
We have to consider the possibility of a terrible mistake, from year 1936 to accept that neutron is not an electron-proton complex of size much larger than proton and with a structure showing spin 1/2. Stereo metric approach demonstrates the balance of electric, strong nuclear and centrifugal forces of nuclei composed by protons.
Dear Matt Strassler I accept that it is dificult to accept this revolutionar concept.
In the references here below, are details of myToE based on stable cyclonic structure of electron (thin ring of large radius) and proton (donut) in ideal gass (old idea of Lord Kelvin), explaining the electric and the nuclear forces as winds created by the two cyclonic eyes (ring and axial) of the particle and the gravity by the diffusion resulting from the pressure gradient.
I am at your disposal for details.
References:
Publication of Physical Theory of Everything by K.S.Papathanassou ISBN 978-960-8257-74-0 Athens, July 2013 and B edition Athens, March 2016
Physical Theory of Everything by K.S.Papathanassou in the publication of the minutes of the 15th Congress of the Greek Physicists Association 9 March 2014
Ιinvestigation of the pending helium cation model by K.S.Papathanassou in the publication of the minutes of the 16th Congress of the Greek physicists Association 18 March 2016
Ιinvestigation of the pending helium cation model by K.S.Papathanassou in the magazine “Physics News” Nr 17 December 2016, of the Greek physicists Association
NVM I GOT IT NOT I REALLY NEED SOME HELP IM DOING HOME WORK !!!!!!!!!!!!!!!!!!!!!!!!!!!!!!!!!!!!!!!!!!!!!!!!!!!!!!! !!!!!!!!!!!!!!!!!!!!!!!!!!!!!!!!!!!!!!!!!!!!!!!!!!!!!!!!!!!!!!!!!!!!!!!!!!!!!!!!!!!!!!!!!!!!!!!!!!!!!!!!!!!!!!!!!!!!!!!!!!!!!!!!!!!!!!!!!!!!!!!!!!!!!!!!!!!!!!!!!!!!!!!!!!!!!!!!!!!!!!!!!!!!!!!!!!!!!!!!!!!!!!!!!!!!!!!!!!!!!!!!!!!!!!!!!!!!!!!!!!!!!!!!!!!!!!!!!!!!!!!!!!!!!!!!!!!!!!!!!!!!!!!!!!!!!!!!!!!!!!!!!!!!!!!!!!!!!!!!!!!!!!!!!!!!!!!!!!!!!!!!!!!!!!!!!!!!!!!!!!!!!!!!!!!!!!!!!!!!!!!!!!!!!!!!!!!!!!!!!!!!!!!!!!!!!!!!!!!!!!!!!!!!!!!!!!!!!!!!!!!!!!!
hi but i NEED help im an 8th grader so can youguys help
Does the proton have less mass because the binding energy is derived form the proton and not the neutron?
Sorry if this question is too basic, but that’s where my physics is.
can anyone tell me that …… a nucleus contains gluons of high electron volts and yet is stable why?????
pls give me the ans as fast as possible
hey how are you;; tell me the reason that proton are packed inside the nucleus into small volume rather than blows a part by electrostic repulsion ? although it is the simple question but give me the very simple answer?
What about what Matt. wrote that at very short distance they will REPEL each other ? is this a change of sign or not ?
No, what it is is less attraction. As an example think of a block of wood bobbing up and down in water. The block has a preferred height above water that is lowest energy (when the block displaces its own weight in water.) If it is too high ( far above the water) it will sink down under gravity, but if it is too low in the water it will move *up*. We can call the force on the block the ‘buoyancy force’, a sort of leftover gravity due to gravity acting on the block and the water (and even the air.)
When it is too low in the water and moving up, has gravity suddenly turned negative on it? No, gravity is always pulling it down, but it is also pulling the water and when the block is too low it is higher energy because some of the water is being pushed up and is pushing back. So a force that is always down produces a leftover force that can be up, down or nothing.
So it is with nucleons; the quarks and gluons that make up the nucleons are tightly bound and nucleons themselves attract each other strongly as well, but when two nucleons are too close the balance shifts, there is still a strong attractive force between the two, but it would be lower energy if they were further apart. The leftover ‘nuclear’ force becomes positive, but at no point does anything ‘change sign’ or charge.
This is common in nature, an electron and proton will not collide in hydrogen as when the electron gets too close it becomes lower energy for it to move away, the sun burns at a near constant rate because if it burns too hot radiation pressure makes it expand, cool and slow down. Some of these cases (mostly those in chemistry) are called ‘dynamic equilibrium. http://en.wikipedia.org/wiki/Dynamic_equilibrium
Since all forces are having non- quantized range of action , how the quantized orbitals / energy levels are related to that forces ? whence comes the discrete effects ?
Put it in another form : what generates the many rules and principles directing the quantum world ? are they necessary or contingent or the result of higher level laws ?
Did you read (newscientist ) magazine on ( crunch time for physics ) where they claim that without new paradigm it is end of the road for physics of sub /ultra realms ?
Do you agree ?
Hi , how are you……
Few points to be clarified , are we now to understand strong force as ( + ) among P and N then ( – ) at very short distance then ( + )among quarks then ( – ) at very short distance ?
What determines electron shells distance from the center ?
Are a/m shells have independent existence even without electrons ? are energy levels having fields of their own ?
What are the relations among particles ripples and particles energy levels ? does the ripple of electron change ( shape , frequency , whatever ) according to its energy / shell ?
thanks
The ‘color force’ between quarks is much more like the electromagnetic force, it doesn’t reverse sign at any distance for example. (Though unlike electromagnetism it doesn’t tend to zero at long distances, resulting in quark confinement.) The strong force is a result of how the ‘color force’ works and as such it is complex in the same way Van Der Waals forces are complex.
The size of electron shells is determined (roughly) by the uncertainty principle. Naively an electron would like to get as close to the proton as possible, however if it did so it would be in an infinitely small volume of space, we would know its position exactly. However, as it is drawn closer to the proton and its position becomes more certain, its speed increases (becomes less certain) Eventually the electron is moving fast enough that it ‘orbits’ the proton in an electron shell. If the mass of the electron were to double, all electron shells would be half their current size. (This is a simplified explanation and the professor discusses it at more length n earlier atom articles.)
Electron shells do not exist without electrons and they most certainly do not have fields of their own. (Recall that protons too are moving, though since they are much more massive they move much less.) Rather the way the universe works is that when an electron and proton meet the electron will emit photons and settle into a stable configuration. You might say that the shells exist ‘potentially’ if anything.
A particle’s properties depend greatly on its situation. Various energy levels are various situations. The property most affected is its ‘shape’ or rather the volume of space it occupies. Various electron shells have different shapes. An electron’s frequency ill decrease as it loses energy, so an electron in an atom will have a lower energy\frequency than free electrons, but this is a very small difference indeed (In hydrogen the electron is 14eV less energetic out of about half a million electron volts of mass.) I am not sure this is the ‘relationship’ you are looking for however.
New Scientist is nice, but their articles tend to speculation and hyperbole. (They have run articles on dismantling the tree of life and gravity radios.) Only time will tell if this is the case with the article you cite.
Excellent Professor, it is Enlightening !
Excellent. We appreciate you time and sacrifice in providing this service.
Neutron is unstable at the distance, shorter than “R” and greater than “R”?. R is the secret of stability of the nucleus and of course the whole of Physics ?
Quantum chromodynamics (QCD) is only a mathematical gauge theory – not a reality(group theoretical property).
The force between quarks is much more stronger than between hadrons(proton, neutron) ?
Residual force could split by nuclear fission, but to split bindind force between quarks will rupture spacetime dimension ? – the energy used to split will induce anti decay(energize new quarks)?
Neutrons and protons have an optimal distance they ‘like’ to stay at. Too close and they will repel, too far and they will attract (far too far and they will ignore each other.) This is because of how the residual strong force works.
The force between hadrons is much, much weaker than the strong force int he same way that the electrical forces between molecules are much weaker than the force holding the electrons to the atoms. (You can break up water molecule force by boiling it, but you must heat steam to thousands of degrees before it starts to break up into nuclei and electrons.)
Quarks can be ‘split apart’ quite easily and this is done regularly by even modest particle accelerators. The problem is however that the ‘split’ always creates more quarks so that everything ends up tucked into neat hadrons quite quickly. If your definition of ‘split’ is to get an individual quark by itself however, then no amount of energy will be enough, it just cannot be done, quarks do not work that way.
The reason that neutrons can be stable inside of nuclei even though they are unstable outside of nuclei is given in this article: http://profmattstrassler.com/articles-and-posts/particle-physics-basics/mass-energy-matter-etc/the-energy-that-holds-things-together/neutron-stability-in-atomic-nuclei/
Would love to hear an explanation of *why* the strong force is so short-ranged. The ranges of the other 3 forces have reasons for their respective ranges which make sense at least some sense to the layman (Inverse Square Law, massive carrier)….but I’ve never been able to understand why the strong force has the range it does. Is there any explanation, or is it just “complicated”?
In a way it is complicated. The big issue is ‘confinement’, where particles that feel the strong force tend to stick together in ‘neutral’ hadrons instead of being free to move through space. This seems to be a result of the force’s carriers (gluons) themselves feeling the strong force. Imagine if photons were electrically charged; (you would need two kinds, each the antiparticle of the other.) Light would repel and attract itself in a complicated mess.
So it is with the strong force. What we see most is the residual, ‘leftover’ force that manages to leak out, and because that remnant is created in a complex way it has complex behavior.
This is true for QCD, but for the nuclear force (ie. the residual strong force) the principal issue is that pions are not massless, no?
The fact that pions are massive is the proximate cause for why the force is short ranged, but *why* are pions so massive? Why do they carry the residual force? Quark confinement is the ultimate reason for there being a residual force in the first place as well as the fact that it is carried by color neutral hadrons.
What issue matters the most depends on what your focus is; the mass of the force carrying particle is a simple explanation for the range of any force, but Tim already understands this and seems to want to know the reason for the precise range of the nuclear force, which I think would require a more technical explanation as to the behavior of virtual pions, their origin and mass.
“*why* are pions so massive?”
Because quarks are massive and so pions are not true NG bosons.
That may be true, but pions are hugely more massive than the quarks they are supposedly composed of, why are they that mass? (If they were as massive as their ‘constituent’ quarks the nuclear force would have at least an order of magnitude more range, greatly altering nuclear physics.) And why can’t quarks themselves or gluons carry the force? Why pions? (And why not say kaons?)
The subject is endlessly interesting, especially when you get into detail.
Well what it comes down to is: the strong force should be just like electromagnetism, but gluons carry colour charge, so what we actually end up with is the residual strong force, carried by pions. This would still be a long-range force if quarks were massless (if their Higgs couping were zero/ the Higgs vev were zero…. however you have it), but since they’re not, the force is short-range. The exact “shortness” can only be characterised relatively, e.g. in comparison to the Bohr radius and what this comes down to is the difference between the electron mass and the QCD scale, for which there is no explanation.
I’m not sure there is any meaningful answer to the question (at least for the time being) for “why are pions that mass?”. They have non-zero mass because QCD has an inbuilt scale, which isn’t predicted by any higher theory.
Interesting, I was under the impression that even if quarks were massless they would still be bound into hadrons. Or would the force be of infinite range, with hadrons simply being infinitely large? I lack understanding on this topic.
James did not say anything that contradicts your impression. Quarks would still be bound in hadrons, even if they were massless. The force due to gluons — the strong nuclear force — would still be short-range. Hadrons would still (in some sense) be of finite size. But the pion, the lightest hadron, would be massless, and it would induce a relatively long-range residual strong force between hadrons. Not so much is known about this force, by the way. My colleagues in nuclear physics tell me that they are not sure what would happen to nuclei in this situation.
I will describe this in some detail when I talk about how protons and neutrons are formed. Kudzu has the right idea but more concepts are needed.
Now I am somewhat confused. According to several posts on this site a large portion of hadron masses comes not from their constituent quarks, but the hoi polloi that is part of their structure, gluons and ‘virtual’ quarks and so on. I assume that even if quarks were massless the bound system would act as if it would have mass, or would the change in hadron structure due to massless quarks mean that this was not the case? What would the proton\neutron mass be, compared to currently?
You are confused, indeed. And it is confusing. Massless up and down quarks would not change hadron masses much at all, EXCEPT for the pion’s mass. The pion is a very special particle, because it is nearly a Nambu-Goldstone boson — and would be exactly a Nambu-Goldstone boson if up and down quarks were massless. This is why the pion is by far the lightest hadron. (Kaons would similarly be massless if strange quarks were massless too. And strictly speaking, the pions would only be precisely massless if the weak nuclear and electromagnetic interactions were turned off.)
How it is possible that a complicated object built from quarks and gluons could be exactly *massless* is one of those magical things about quantum field theory that tells you that the world is more subtle than it appears. I have not attempted to explain this point anywhere on this website.
In a way it is easy to show why pions are massless, if you don’t look inside them too carefully. Nambu-Goldstone bosons arise in classical field theory with a tiny bit of quantum mechanics added. But to understand their internal structure? That’s harder…
This answer is satisfactory (Though I would like to hear more on the pion’s mass, which now you point it out is incredibly light.) It makes a lot of sense, once you wrap your head around some of the details. (I found an interesting lecture related to the subject here: http://www.youtube.com/watch?v=8OuSnlVyriU that was enlightening on some points (and deeply confusing on others.))
Many thanks for your replies.
Another interesting article. I am however confused by the ‘accident’ of a narrow range of stable nuclei; I was always told this was due to the fact that neutrons are nearly stable (being only slightly more massive than a proton and electron.) if they were very unstable they would decay in all nuclei and only protons would remain, whereas if they were stable even pure neutron nuclei would be favorable. Or is it the neutrons near stability that is the accident?
I’ve gotten a couple of questions about this and am now second-guessing myself. Unfortunately I’m overworked right now — will come back to this when I can…
Well, what I find in explicable is the repulsive character of the strong force at very short ranges. The strong force is attractive at all distances less than 3fm, except for the discontinuity of repulsion between 0 and .5fm. inside the nucleon it is strong again thus confinement. I guess it is good for the universe that this is so, but I have never heard an. Explanation for it. Seems very peculiar behavior.
Hi Matt, glad to see you are still finding some time to continue with your excellent series of on-line articles. I suppose your comments about the relative weakness of the electromagnetic force also applies to the weak force (or electroweak force as we are supposed to call it now). However I understood it is the weak force that controls what used to be termed radioactive “beta” decay whereas presumably the strong force controls what used to be called radioactive “alpha” decay? I have a feeling you are coming to that so don’t bother to reply directly if this is coming soon.
Matt, thank you as always. In one of the later articles, can you please include an explanation of the energy change exposed by fission/fusion and atomic mass not being exactly linear to the numbers of Protons & Neutrons. Naively, on side there is one form of potential energy, mediated by packing and/or screening, but why does extra stability get reflected by a mass/energy change, rather than eg just stronger bonds?