Matt Strassler 10/19/11
Another experiment has weighed in on superluminal neutrinos — indirectly. The ICARUS experiment has measured the energy spectrum of neutrinos traveling from CERN to Gran Sasso. They confirm that the Cohen-Glashow effect, which I described in some detail here, is not occurring in the beam of neutrinos that OPERA is also using. The distribution of neutrino energies in the beam is undistorted. If standard Cerenkov processes take place, then these neutrinos are not traveling faster than light.
At least one blogger has said this refutes the OPERA experiment. Hmmm… for my part, I don’t think it really changes the situation much at all. It only checks something that we were already pretty sure of. As I explained in my post on the subject, Cohen-Glashow emission would have had a big effect on the OPERA neutrino beam, one that OPERA itself would have detected. That was Cohen and Glashow’s main point. OPERA didn’t see any hint of such an effect. All ICARUS has done, as far as I can tell, is confirm that indeed there’ s no such effect, to significantly higher precision. If you want to say that ICARUS has refuted OPERA, you really have to say that OPERA refuted itself first… that the experiment was self-contradictory.
What’s the loophole? It’s small, but it’s still there. We already knew, both from Cohen-Glashow emission and from the calculation of Giudice et al. that OPERA almost certainly cannot be right unless relativity is altered in such a way as to shut off Cerenkov-like processes at high energy. That loophole may well be too narrow for any decent modification of relativity to get through. But I don’t think ICARUS’s result changes the location or nature of the loophole.
UPDATE: In a question below it was asked whether sterile neutrinos [new types of neutrino-like particles that aren’t affected by the weak nuclear force and therefore would not have large Cohen-Glashow emission] could also pose an acceptable loophole, by oscillating with ordinary neutrinos. I can’t make a clean argument against it without going a calculation, so for now I have to say that, though I’m dubious, I am not sure that loophole is closed yet.
UPDATE: I hadn’t had time when I first put this post up to explain why ICARUS and OPERA are measuring the same neutrino beam. Both experiments are in the Gran Sasso lab, quite near each other. By the time the neutrinos arrive at Gran Sasso, the beam of neutrinos is quite spread out — a couple of kilometers wide, I believe — so the Gran Sasso experiments are sitting in the same neutrino beam. We can expect other Gran Sasso experiments to weigh in with their own observations over time.
UPDATE: Here’s a picture comparing my sketch (from my post ten days ago on Cohen-Glashow emission) of what CG emission predicts for the OPERA beam (an effect that OPERA itself would have seen) to the measurement from ICARUS. Note the peak and the tail, marked by the black arrow and violet arrow on both plots; if Cohen-Glashow emission were operating, the tail would be gone and the peak would have shifted to the left.
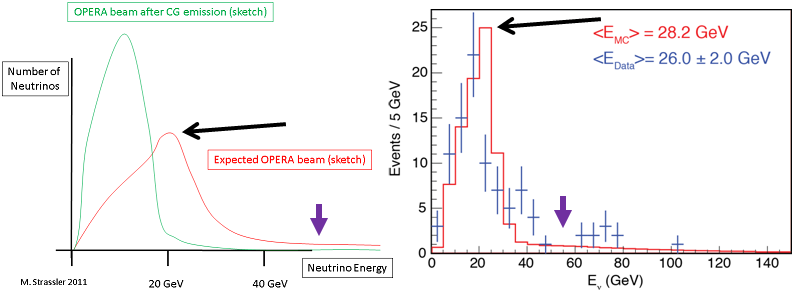
Comments welcome.
2 Responses
How do they measure differences in billionths of a second in opera
Well — hmm — measuring times in billionths of a second is fairly routine with modern electronics. Any of Intel’s chips, for example, does calculations a little bit faster than once every billionth of a second (one gigahertz frequency, or equivalently once a nanosecond). 100 picosecond timing (0.1 nanosecond) is not that unusual, and 10 picosecond timing is just beyond the state of the art in particle detectors. The electronic signals moving around in the LHC detectors have to be synchronized to somewhere between a few nanoseconds to better than a nanosecond. A proton-proton collision occurs in each LHC experiment every 50 nanoseconds (and the machine was designed to operate eventually with 25 nanoseconds between collisions). So this isn’t the hard part of OPERA.
Now if you want a layperson-friendly and accessible account of how nanosecond timing is actually done, I’m not the right guy to provide it. One of my experimental colleagues who teaches electronics would give a much better answer. Readers who know how to explain this properly are welcome to answer, or to suggest a suitable website.
My (imperfect!) understanding is that the real challenge with the timing at OPERA is making sure all possible time delays as signals move down wires are properly accounted for. They also have to ensure that the clocks at CERN and OPERA are synchronized to sufficient accuracy, but they use two independent methods (GPS timing, and atomic clocks moved from one lab to the other) that give the same answer. Finally of course one needs to account for any subtle effects of relativity.