Greetings from Geneva, and CERN, the laboratory that hosts the Large Hadron Collider [LHC], where the Higgs particle was found by the physicists at the ATLAS and CMS experiments. Between jet lag, preparing a talk for Wednesday, and talking to many experimental and theoretical particle physicists from morning til night, it will be a pretty exhausting week.
The initial purpose of this trip is to participate in a conference held by the LHCb experiment, entitled “Implications of LHCb measurements and future prospects.” Its goal is to bring theoretical particle physicists and LHCb experimenters together, to exchange information about what has been and what can be measured at LHCb.
On this website I’ve mostly written about ATLAS and CMS, partly because LHCb’s measurements are often quite subtle to explain, and partly because the Higgs particle search, the highlight of the early stage of the LHC, was really ATLAS’s and CMS’s task. But this week’s activities gives me a nice opportunity to put the focus on this very interesting experiment, which is quite different from ATLAS and CMS both in its design and in its goals, and to explain its important role.
ATLAS and CMS were built as general purpose detectors, whose first goal was to find the Higgs particle and whose second was to find (potentially rare) signs of any other high-energy processes that are not predicted by the Standard Model, the equations we use to describe all the known particles and forces of nature. Crudely speaking, ATLAS and CMS are ideal for looking for new phenomena in the 100 to 5000 GeV energy range (though we won’t reach the upper end of the range until 2015 and beyond.)
LHCb, by contrast, was built to study in great detail the bottom and charm quarks, and the hadrons (particles made from quarks, anti-quarks and gluons) that contain them. These quarks and their antiquarks are produced in enormous abundance at the LHC. They and the hadrons that contain them have masses in the 1.5 to 10 GeV/c² range… not much heavier than protons, and much lower than what ATLAS and CMS are geared to study. And this is why LHCb has been making crucial high-precision tests of the Standard Model using bottom- and charm-containing hadrons. (Crucial, but not, despite repeated claims by the LHCb press office, capable of ruling out supersymmetry, which no single measurement can possibly do.)
Although this is the rough division of labor among these experiments, it’s too simplistic to describe the experiments this way. ATLAS and CMS can do quite a lot of physics at the low mass range, and in some measurements can compete well with LHCb. Less well-known is that LHCb may be able to do a small but critical set of measurements involving higher energies than is their usual target.
LHCb is very different from ATLAS and CMS in many ways, and the most obvious is its shape. ATLAS and CMS look like giant barrels centered on the location of the proton-proton collisions, and are designed to measure as many particles as possible that are produced in the collision of two protons. LHCb’s shape is more like a wedge, with one end surrounding the collision point.
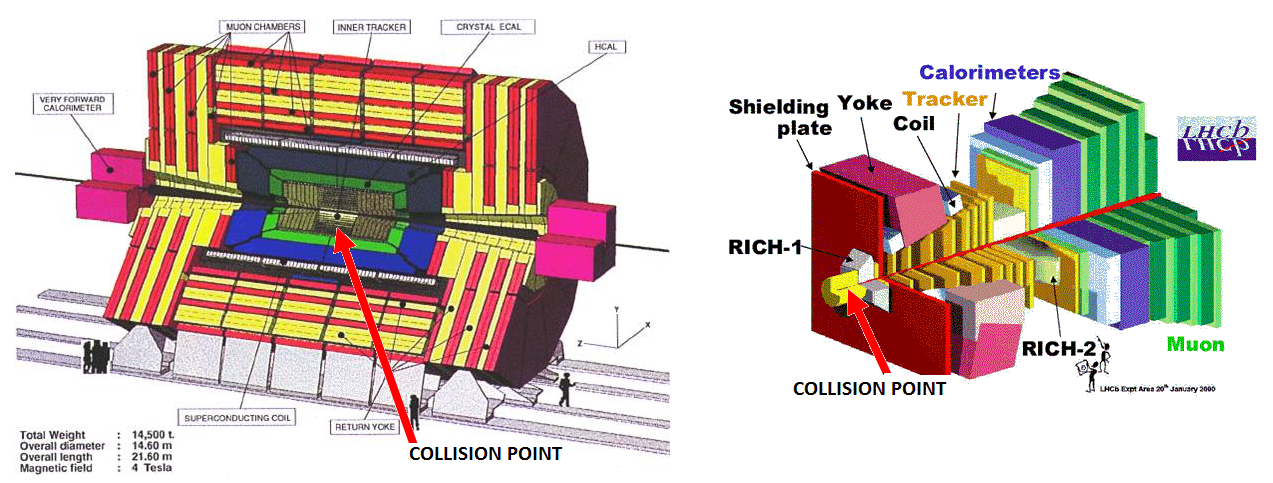
This shape only allows it to measure those particle that go in the “forward” direction — close to the direction of one of the proton beams. (“Backward” would be near the other beam; the distinction between forward and backward is arbitrary, because the two proton beams have the same properties. “Central” would be far from either beam.) Unlike ATLAS and CMS, LHCb is not used to reconstruct the whole collision; many of the particles produced in the collision go into backward or central regions which LHCb can’t observe. This has some disadvantages, and in particular put LHCb out of the running for the Higgs discovery. But a significant fraction of the bottom and charm quarks produced in proton-proton collisions go “forward” or “backward”, so a forward-looking design is fine if it’s bottom and charm quarks you’re interested in. And such a design is a lot cheaper, too. It also means that LHCb is well positioned to make some other measurements where the forward direction is important. I’ll give you one or two examples later in the week.
To make their measurements of bottom and charm quarks, LHCb makes use of the fact that these quarks decay after about a trillionth of a second (a picosecond) [or longer if, as is commonly the case, there is significant time dilation due to Einstein’s relativity effects on very fast particles]. This is long enough for them to travel a measurable distance — typically a millimeter or more. LHCb is designed to make the measurements of charged particles with terrific precision, allowing them to infer a slight difference between the proton-proton collision point, from which most low-energy charged particles will emerge, and the location where some other charged particles may have been produced in the decay of a bottom hadron or some other particle that travels a millimeter or more before decaying. The ability to do precision “tracking” of the charged particles makes LHCb sensitive to the presence of any as-yet unknown particles that might be produced and then decay after traveling a small or moderate distance. More on that later in the week.
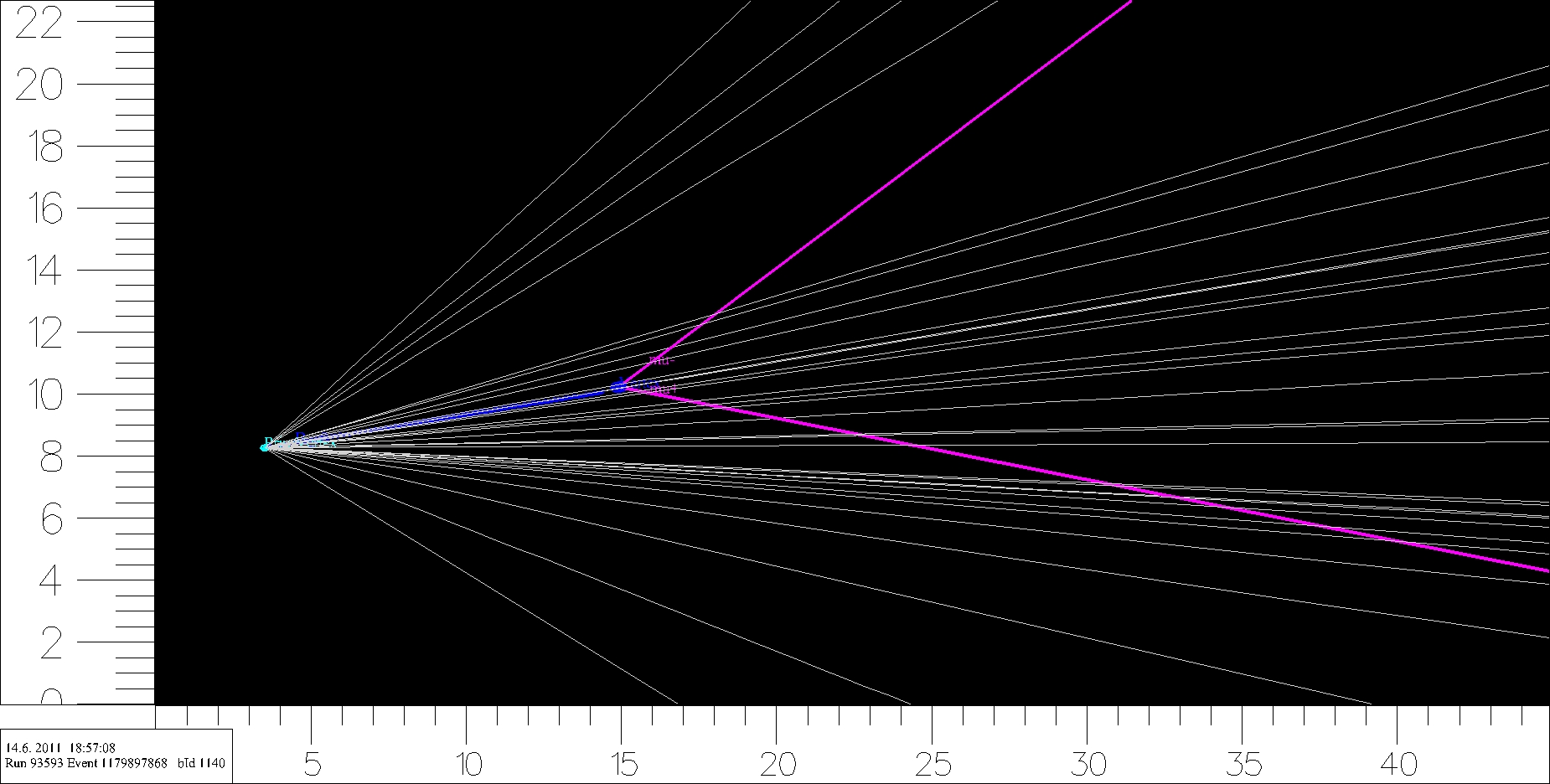
One other thing to know about LHCb; in order to make their precise measurements possible, and to deal with the fact that they don’t observe a whole collision, they can’t afford to have too many collisions going on at once. ATLAS and CMS have been coping with ten to twenty simultaneous proton-proton collisions; this is part of what is known as “pile-up”. But near LHCb the LHC beams are adjusted so that the number of collisions at LHCb is often limited to just one or two or three simultaneous collisions. This has the downside that the amount of data LHCb collected in 2011 was about 1/5 of what ATLAS and CMS each collected, while for 2012 the number was more like 1/10. But LHCb can do a number of things to make up for this lower rate; in particular their trigger system is more forgiving than that of ATLAS or CMS, so there are certain things they can measure using data of a sort that ATLAS and CMS have no choice but to throw away.
5 Responses
i have doubts that photons travelling forward and backward have the same property,because particles and antiparticles are differents travelling in backward and forward in the spacetime because the operator PT and CP are not totally conserved
I think “forward” and “backward” here are equivalent directions, except that the detector is only on the “forward” side. Reading carefully, I think they could have switched directions and still had the same setup.
I agree. Remember, these are identical colliding proton beams. There might be slight difference because they do not collide at exactly 180 degrees, but at slightly different angle. I could be wrong of course!!