I’ve been busy with some pressing work in service of the triggering strategy at the Large Hadron Collider [LHC] experiments for the last few days… (and if you understand what the trigger does, you know that stuff having to do with triggering pretty much takes priority over almost anything else, including sleep.) So my apologies that I’ve been a little slow to sum up this week’s updated results on the search for the Higgs particle. Today I hope to make amends.
In Tuesday’s post I reported that the ATLAS and CMS experiments at the LHC had updated their preliminary results on the Higgs search presented on December 13th, through the release of documents intended for publication [so-called “preprints,” intended for submission to a journal for peer review.] In updates to that post, I highlighted two issues which I found particularly interesting in comparing the updated information to the presentations in December. The first of these represents additional evidence from CMS, which strengthens their case for a signal of a Higgs-like particle with a mass around 124 GeV/c2. The second of these involves the lack of any improvement in the concordance between ATLAS and CMS, which one might have hoped for, and in whose absence the results still remain almost as inconclusive as they were back in December. Today I want to explain these in a bit more detail.
The New Result From CMS
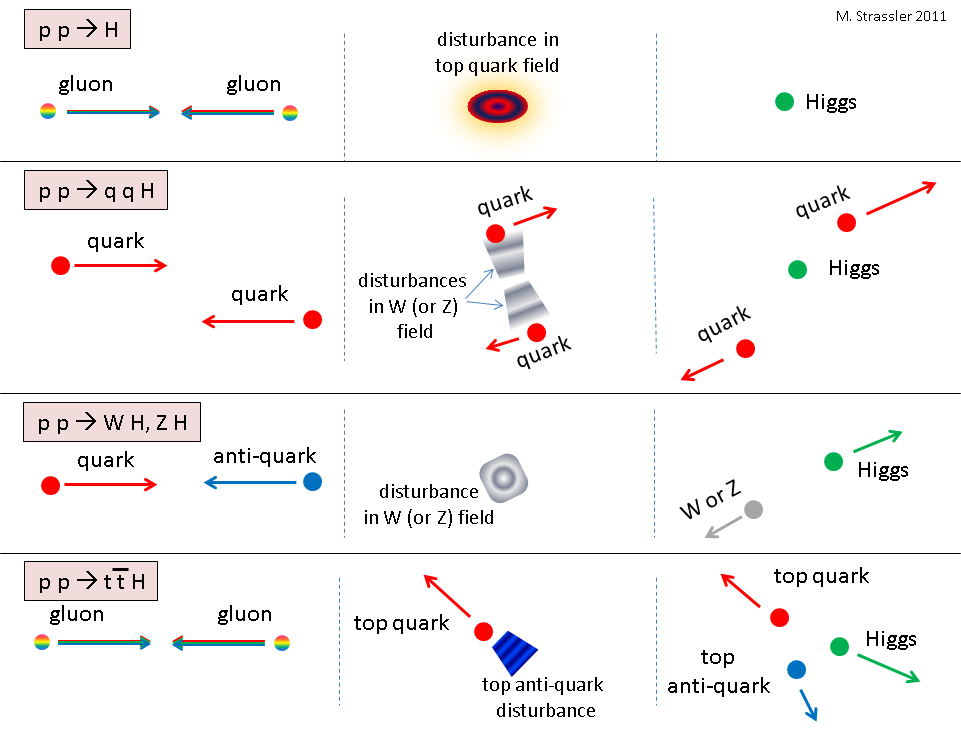
CMS, like ATLAS, has been searching for Standard Model (or Standard-Model-like) Higgs particles decaying to two photons, independent of how they are produced. [Recall that the “Standard Model Higgs particle” is the simplest form of Higgs particle that might be present in nature.] But there are several ways to produce Standard Model Higgs particles (see Figure 1), the two largest of which are (1) g g –> H, two gluons (one from each proton `p’, so this is also called p p –> H) colliding to make a Higgs particle, and (2) q q –> q q H, two quarks scattering off each other and producing W and Z “virtual particles” (disturbances in the W and Z fields which aren’t particles at all), which meet in the middle and fuse to create a Higgs particle. The rate at which q q –> q q H occurs is about 10-15 times smaller than the rate for g g –> H. Now if there is a Standard Model Higgs particle at around 125 GeV/c2, the number of these Higgs particles already produced in ATLAS and CMS (each) and decaying to two photons is about 150 or so via g g –> H, and about 10 or so produced in q q –> q q H. Not all of these would be detected, so the numbers observed would be somewhat smaller.
Let me start by making a simple and naive (and wrong) comment about these two production processes. The conclusion we’ll draw from the naive viewpoint will turn out to be correct, but the details will change, in an important way, when I correct these initial statements toward the end of this section.
From Figure 1, you can see that the thing which makes q q –> q q H different from the g g –> H process is that there are two outgoing quarks, which turn into two outgoing jets (sprays of hadrons). In other words, what the experiments will observe is collisions with two photons (from the decaying Higgs) along with two jets. So (naively) they should be able to look for the q q –> q q H process and measure it separately from the g g –> H process by separating the events with two photons into those that have two jets and those that don’t.
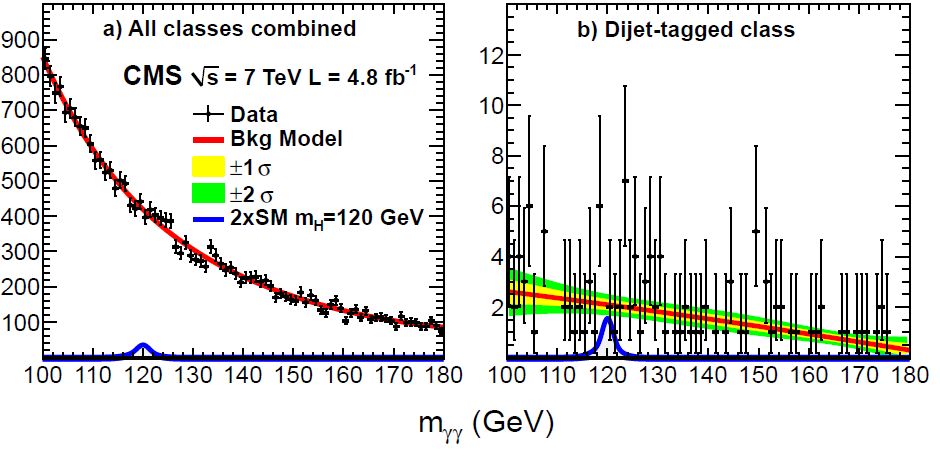
Now why would that buy you anything? Because the backgrounds (processes that mimic a Higgs signal but have nothing to do with a Higgs at all) to these two classes of events are very different. In particular, although the signal for q q –> q q H is about 10 or so times smaller than that of g g –> H, the background is a lot smaller, by a factor of 100. Roughly speaking, in the g g –> H case one is looking for tens of events on a background of many hundreds, while in the q q –> q q H case one is looking for a few events on a background of a few.
This is clear from the CMS results, which are shown in Figure 2. On the left is the invariant mass of the two photons in all observed collisions with two photons. That includes any generated either by g g –> H or q q –> q q H. However, the majority of the events in any bin are due to background (collisions that generate photon pairs for reasons that have nothing to do with the Higgs particle. ) You can see that the size of any expected excess from a Standard Model Higgs (half the size of the blue bump at the bottom of the plot) is very small compared to the sheer number of events above the bump. That’s why you have to look really closely to see the small excess that CMS claims to observe, running from 121 to 126 GeV and centered around 123-124 GeV. The background is hundreds of events per bin, and the expected signal is just a few dozen per bin.
On the right of Figure 2 is the same plot for those collisions that (roughly — see below) have two jets and two photons. You see that the background is about 2 events per bin, and the expected signal is one or two events per bin. The fact that the number of background events per bin is really small means that statistical fluctuations, bin to bin, are large; that’s why the data is all over the place on the right-hand plot. But you can see by eye that there are some extra events in the 123-124 GeV bin. Now you have to be a little careful; we don’t expect all of the signal events to be in one bin. They should be spread out over three or four, because the experimental measurement, though precise, still has its limitations. So you should look at the three or four bins around 124, and ask if you see extra events. And you do — more than you would expect from a Standard Model Higgs particle, in fact.
Now the statistical significance of the excess isn’t very large. But if the small excess seen on the left-hand plot of Figure 2 were a pure statistical fluctuation of the background, there would be no particular reason for the small fraction of these collisions that appear in the right-hand plot of Figure 2 to show an excess in the same place. On the other hand, that is exactly what you would expect if there were a Higgs particle of Standard Model type at this mass. So the observed excess in the right-hand plot makes the CMS case for a Higgs particle — which was a circumstantial case involving several different measurements with very little evidence on their own — somewhat stronger. Although the statistical significance of the combination of all their measurements doesn’t change much, the statistical significance of the part of the signal that I feel most comfortable trusting — the part that comes from the two-photon search and the four-lepton search — has certainly increased.
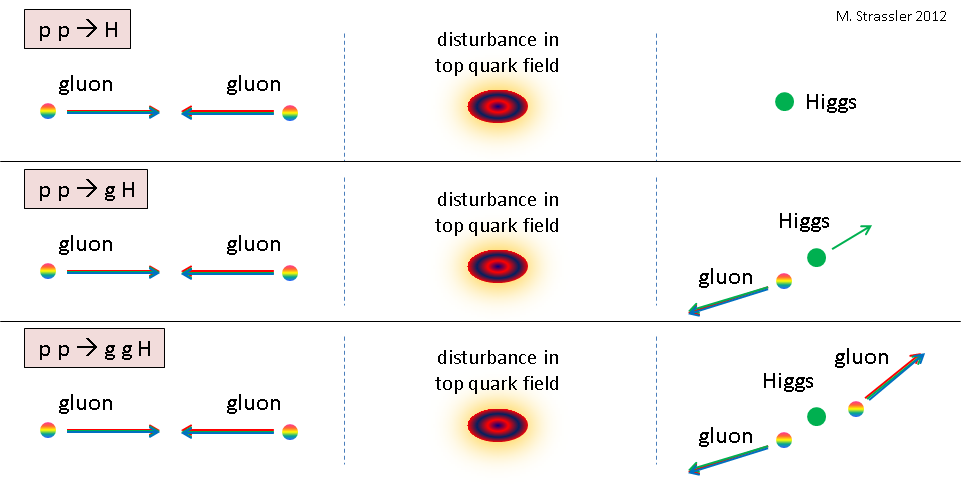
Ok: let me correct the naive aspects of what I’ve told you. Simply requiring two jets is not nearly enough to separate g g –> H from q q –> q q H, because there is a certain probability that a gluon or two will be produced in g g –> H. In other words, there is a chance that rather than merely being g g –> H, the collision of two gluons will lead to g g –> g H or g g –> g g H. (See Figure 3.) Gluons, quarks and antiquarks all make jets, and gluon jets look a lot like quark jets and cannot typically be distinguished. So g g –> g g H looks a lot like q q –> q q H. Now there are tricks to try to separate these processes to a degree (involving a requirement on where the jets and photons are actually heading relative to one another) but (a) these tricks only allow partial separation of q q –> q q H and g g –> g g H , and worse (b) the ability of theorists to calculate how well the tricks work in separating the two is very limited. The CMS experimenters assign a 70% systematic uncertainty to how much g g –> g g H remains after they play their tricks, but I can easily imagine this is a significant underestimate. I would like to know more about how they came up with this number; if someone told me the systematic uncertainty was twice this big I would not flinch, given how intricate their tricks for selecting events are, and how little I would trust existing theoretical calculations in that context. We need to hear from the world’s experts on these calculations, and see if they agree with each other that this 70% number is large enough.
If my concerns were valid, what that would mean is that once you play these intricate tricks (as was done for the plot on the right side of Figure 2) you would be left with a very uncertain theoretical prediction for exactly how many events a Standard Model Higgs particle would give you. The error bar on the theoretical prediction would probably be a factor of 2, possibly worse. And if I were right, CMS might then be underestimating the Standard Model prediction, leading them to over-interpret their result in Figure 2 as additional evidence for an excess above Standard Model expectations.
Indeed there has been some excitement among some of my theoretical colleagues about this suggestion that the number of events observed by CMS is larger than would be expected from a Standard Model Higgs particle. This is because the number of events at ATLAS also seems to be larger than expected. But aside from the fact that the number of events in the excess seen in the right-hand plot is very low (and therefore subject to large fluctuations: if you expect 2 or 3 events, the statistical probability of getting 8 is not as small as you would think), the expectation is itself quite uncertain (and thus perhaps the 2 or 3 events expected should actually be 5 or 6.) And there’s another reason — see below — to be suspicious of any excess above the Standard Model at both ATLAS and CMS. So I would be very, very cautious about reading anything into the larger number of events. Of course it is intriguing and fun to think about, but it is far too early to get excited.
In particular, to my excited colleagues: let’s recall that over the years the rate for the process g g –> H changed by a factor of 3 as theorists did more accurate calculations. What do we currently know about the process g g –> g g H, especially in this very limited kinematic region, given that it has been calculated only to leading order in perturbation theory? Even if there *is* an excess above the expectations for a Standard Model Higgs in CMS’s data, we could perhaps just as well interpret it as an excess in g g –> H only, along with an underestimate of the contribution of g g –> g g H to this kinematic region, and with NO excess in q q –> q q H at all.
Why don’t CMS and ATLAS two-photon results line up better?
Now, let’s talk about the second issue: the fact that there was no improvement in the discrepancy in the preferred mass for ATLAS and CMS’s excesses (if interpreted as due to a new particle.) The inconsistency isn’t so large as to make the measurements clearly contradictory, but neither is it small enough that one can ignore it.
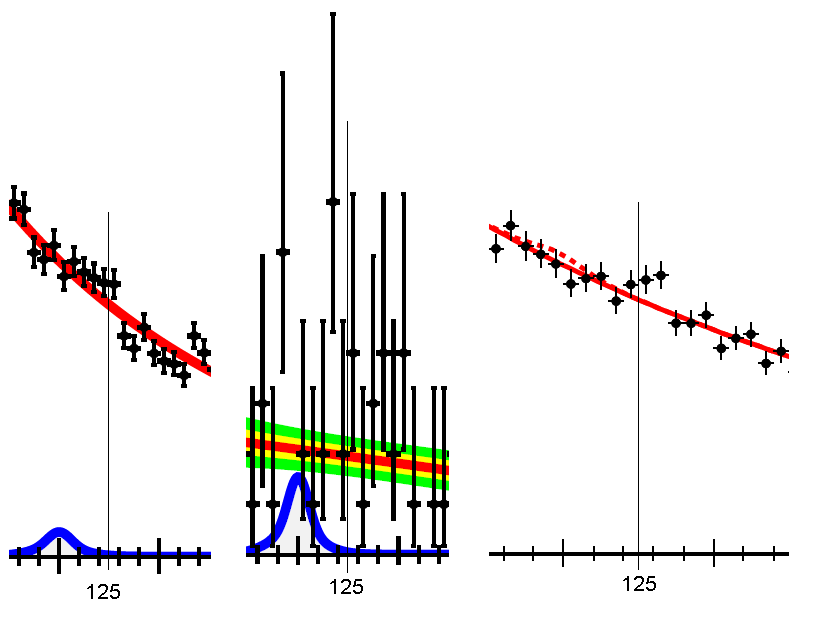
First, let me bring your attention to the discrepancy; in Figure 4 I’ve pulled out the region from 115 to 135 GeV for the left and right plots in Figure 2, and put them next to an excerpt from ATLAS’s most recent plot covering the same region. Note the line that I have drawn on all three plots dividing events above 125 GeV from events below 125 GeV. What you notice is that ATLAS’s largest excess (126-127) is at a point where CMS has a deficit, and that ATLAS has a deficit across some of CMS’s excess. I do not want to overstate the importance of this observation; it may easily be a consequence of small statistics. But neither should it be understated. If anyone tells you that the case for the Higgs is firm, ask them about this discrepancy, which (when the case actually does become firm) had better go away.
There are three possible reasons for this discrepancy.
- There is no Higgs signal at all; ATLAS is seeing some fluctuations in its data, and separately, CMS is seeing some fluctuations in its data. By chance they happen to be close together, but the lack of consistency is reflective of the fact that they are actually independent effects that have nothing to do with each other.
- There is a signal, but the shape and location of the signal are distorted because either ATLAS’s or CMS’s signal peak is sitting on top of a large background fluctuation. For example, this could easily explain why ATLAS has a peak that is larger and narrower than expected from a pure Standard Model signal, an example of which (the dotted red line centered around 120 GeV) is shown on the ATLAS plot at right in Figure 4. Were this true, by the way, then the excess at ATLAS would be a signal plus a background fluctuation, which combine to give an excess that seems too large for a Standard Model Higgs, but which will return toward the expected size as more data is gathered and the fluctuation in the background is smoothed away.
- There is a signal, and either ATLAS or CMS has its photon energy measurement wrong by 1-2%. Calibrating photon energies is not easy, and the mass measurement needs to be accurate to about 1%. I certainly thought it was possible that when the preliminary results of December turned into preprints we’d see a small shift in the mass measurement, up or down, at either CMS or ATLAS, one that might move their two-photon measurements together (or apart) by as much as 1 GeV/c2 or even more. It appears we did not. The preprints show essentially no difference in the two-photon distributions compared to the Dec. 13th presentations. And this suggests that the experiments are reasonably confident in their energy measurements. That said, it appears that when the two-photon measurements are combined with the four-lepton measurements, the result for ATLAS and the result for CMS, whose preferred values were separated by about 2 GeV/c2, have now moved slightly further apart, by less than half a GeV/c2. [I think this is due to a shift in the four-lepton measurement of ATLAS, but I could be wrong; I haven’t been able to fully track this down yet.]
I don’t know how to evaluate which of these possibilities is most likely, and even if I did, the truth doesn’t care what I (or you) think. We’ll just have to wait and see.
Summary of the Update
To sum up: the finalizing of the preliminary results from Dec. 13th has had the following effects (so far)
- The CMS excess at 123-124 GeV/c2 in events with two photons and two jets means a moderate but notable improvement in the credibility of their case, which previously relied on combining a small excess in two photons with a very small excess in four other measurements. The case is more robust now. Meanwhile the preferred value of the mass has drifted just a tiny bit higher.
- The ATLAS result is essentially unchanged, though the preferred value of the mass has drifted a bit higher.
- The discrepancy between the preferred masses for the ATLAS and CMS measurements has slightly increased, not enough to cause a reevaluation of the situation, but eliminating any possibility that they might in the near term shift in such a way to become more consistent. Consistency (or clear inconsistency) will have to await a lot more data.
- Both experiments appear to see excesses that are larger than would be expected for a Standard Model Higgs particle of this mass, by perhaps as much as a factor of 2, but given the large statistical uncertainties and (for the most recent CMS result) large systematic uncertainties, one should not be surprised if all such indications disappear over time.
The excesses in the measurements remain, within the uncertainties, consistent with a Standard Model Higgs, or something rather similar, of around 125 GeV/c2. Due to the new CMS result, I am somewhat more optimistic that this is a real signal than I was in December, but we still have a long way to go before this all begins to settle down.
10 Responses
I love your blog; however, I cannot read it because the “Follow” block, blocks my view of the words! I have already signed up to follow “Of Particular Significance! How do I get rid of the effing block?
Really! Thanks for letting me know. What are you using to try to read it? A computer browser, and if so which one? Ipad? Cell phone?
Matt, maybe I am just dense, I was a bit confused by your opening statement “The mechanisms by which a Standard Model Higgs can be produced, from largest to smallest” – by largest to smallest mechanism do you mean probability as a mechanism of filtering results? size of the experiment as in the physical mechanism? number of resulting particles as in the mechanism for identifying the Higgs? Or something else??
E a r l
Thanks for asking; I always appreciate questions seeking clarity in my wording. “Largest” means the largest production rate (i.e. this is the way most Higgs particles are produced at the LHC). I’ve adjusted the wording in the text to clarify the point. Please let me know if you think any ambiguity or potential confusion remains.
Atlas Collaboration Feb. 8, 2012 paper
http://arxiv.org/abs/1202.1636
CMS Collaboration Feb 7, 2012 paper
http://arxiv.org/abs/1202.1488
Nice update. A naive person would add the CMS and ATLAS distributions together and the dip in CMS would just about null the excess in ATLAS. But, as Richard Nixon said (but was deleted by Rosemary Woods)… that would be wrong.
Hi Matt,
You probably should have looked into this plot as well:
https://atlas.web.cern.ch/Atlas/GROUPS/PHYSICS/PAPERS/HIGG-2012-02/figaux_27.png
Best,
When you say “should” – is there something specific you have in mind? I don’t feel that I have much useful to say about this plot; the 9 different classes of diphoton events in the ATLAS analysis are organized by where and how the photons appear in the ATLAS detector, and I have no special insights into that. [This is in contrast to the CMS di-jet tagged sample, which is organized by the presence and kinematics of additional jets.]