It’s a lot easier to map the Earth than it used to be. Before satellites, you had to do many careful measurements of distances and directions, at many different locations around the world, and combine them all to build a picture of a world you couldn’t see. That’s part of why maps and globes made in past centuries had so many inaccuracies and distortions; it was a tough business.
How that changed in the 1960s! The first full photograph of the Earth that I’m aware of was made in 1967 by the ATS-3 satellite (were there earlier ones?) So much simpler… the whole planet laid out in front of you. You just need a few photographs like this, and the era of measuring from one point on the ground to another is mostly over.
But the challenge of trying to measure things when you’re stuck within them, and can’t step outside them, hasn’t gone away. Just as we could see in telescopes that the Moon and Mars are ball-shaped, long before we could observe the Earth itself, today we can see other galaxies in great detail, but we still struggle to build a complete picture of our own, the Milky Way. The Gaia satellite is trying hard.
To determine the Earth’s two-dimensional surface is really round took some clever thinking. Aristotle, in ancient Greek times, noted that the Earth’s shadow on the Moon during a lunar eclipse is always curved in the same way — it doesn’t matter what time of day or year the eclipse occurs, or whether the shadow is on the north, east, west or south side of the Moon. This feature is to be expected if the Earth’s a ball, like the Moon and Sun, and very difficult to explain otherwise. [Try to figure out what you might see if it were cylinder-shaped!]
But there are other tricks you can use if you have a hunch that the place you live on, or in, is of finite size.
One Dimension: the Possibly Circular Canal
Suppose you live on the banks of a canal, a long thin channel extending off to the horizon, like a river without any flow. And suppose you suspect that this canal forms a loop, surrounding a large island. How could you check? Well, if you had a boat, you could row yourself down the canal; or you could walk along the shore. If the canal is really in the shape of a loop, you’ll eventually come back to your starting point. But maybe you’re worried such a journey would be too long, difficult, risky, expensive. Do you have other options?
Here’s one: suppose you could make a big wave moving in the clockwise direction around the canal. The wave, unlike you, wouldn’t need any food and drink or fuel for the journey — so time and money would not be a problem. The wave would move down the canal at a definite speed [I’m assuming here that it maintains its height], and if the canal were really a loop, then after some time T you’d see the wave return, still moving clockwise, and pass by you. If you waited the same amount of time T again, you’d see the same wave a second time, again clockwise. After the same amount of time T, you’d see it a third time.
If instead the canal were a finite strip, then the wave would reflect off the end, and so the wave would return from the opposite direction. If it were infinite in length, it would never return. And if it had a complicated shape — perhaps a P or an R or a B instead of an O — you would get multiple waves in a complex pattern. But the simple pattern in which the waves return again and again, from the same direction, after a time T, is consistent with the canal being a simple loop.
You could try sending a wave counterclockwise too, and you’d expect the same pattern if the canal’s a loop.
As the wave passes you, you can also estimate its speed v. Having also measured T, you can now determine the length L of the canal. It’s the wave speed times the time T for the wave to go round once:
- L = v T
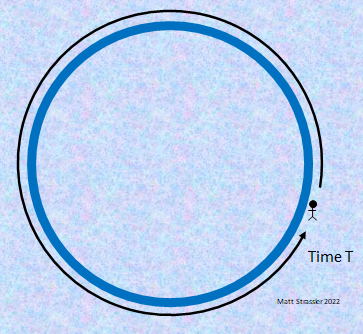
Perhaps making such a wave is too difficult for you, but if you’re lucky, someone or something down the canal may make a giant splash. Then you’ll see the ripples from the splash come by in a similar pattern. Now waves will travel both counterclockwise and clockwise around the canal, and they probably won’t arrive at the same time. That doesn’t matter, though. You’ll see the clockwise waves repeat after a time T, and you’ll see the same for the counterclockwise waves. Seeing both of them repeat after the same time T will give you confidence that the canal’s really a simple loop
To be specific, let’s call t1 the time you measure the first wave, t2 the second wave, t3 the third, t4 the fourth, and so on; if the first wave is counterclockwise, then the second is clockwise (see Figure 2), the third counterclockwise, and so on. (This won’t be true if instead of a loop the canal is in the form of a line segment! A reflection off the end could make the first two waves come from the same direction.) As the clockwise waves will repeat after a time T, and the same for the counterclockwise waves, it will be the case, if the canal’s a loop, that
- t3 – t1 = t4 – t2 = T
- L = v T
There’s more; if you know the time ts when the splash happened and you know the wave speed, then you can learn how far away the splash was from you:
- D = v ( t1 – ts )
But even if you don’t know what time the splash happened, you can figure it out; see Figure 2. The distance traveled by the counterclockwise wave to get to you, plus the distance traveled by the clockwise wave to do the same, equals the full distance round the circle (Figure 2), so the time that the counterclockwise wave required to reach you ( t1 – ts ) plus the corresponding time for the clockwise wave ( t2 – ts ) must be equal to T.
- T = ( t1 – ts ) + ( t2 – ts ) = t1 + t2 – 2ts , which implies ts = 1/2 (t1 + t2 – T)
If you look closely at these four bold-faced equations, they tell you that you can determine T, L, D and ts , properties of the loop and the splash, if you know t1, t2 and t3 and v, which are all things that you can measure without going anywhere. From this point of view t4 is a bonus, a nice check that things are working as expected.
Even better, if you have a friend down the canal who makes the same measurements, that friend won’t get the same answers for t1, t2, t3 and t4 ; the waves arrive at different times for your friend than for you. But when you obtain T and L and ts from the waves you see, and your friend does the same, you’d better get the same answer — because these are properties of the loop and splash, and don’t care where either you or your friend is located.
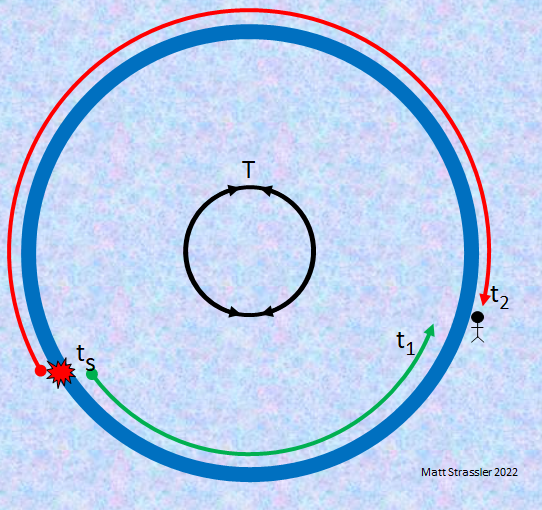
By themselves, these equations do not prove the canal is round, though they are consistent with it. They only tell you that it’s a loop of length L, with no kinks which could cause extra reflections. Still, it’s a lot of information for a very low price, without taking a boat around the loop, walking all around it, or sending up a drone to take a photograph. The waves have done all the work for you.
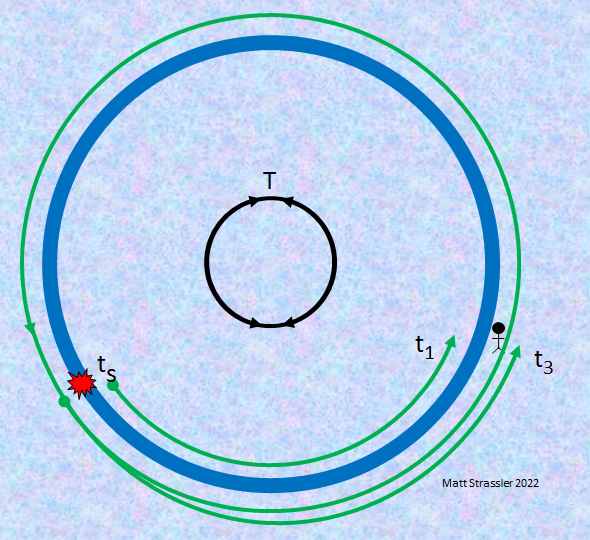
Two Dimensions: the Possibly Round Surface of the Earth
What would be different if you lived on a sphere? (A subtlety of language: by “sphere,” I do not mean “ball”, which is three-dimensional; I mean the surface of the ball, which is two-dimensional. In this terminology, the Earth is a ball, while its surface is a sphere, approximately.) Again, of course, you always have the option of traveling round the sphere yourself and exploring it, checking that no matter what direction you go in, if you walk in a perfectly straight line, you will always come back to your starting point after you travel the circumference of the sphere. But that’s expensive and time-consuming and not very practical. What other options do you have?
You could wait for a big splash in the atmosphere — a natural one like a volcanic eruption, or an artificial one of similar size (fortunately now forbidden by nuclear testing treaties). This opportunity, if you want to call it that, came this past week, unfortunately near an inhabited area and at the ocean’s surface within the Kingdom of Tonga, with ensuing loss of life, as well as the destruction of crops and homes; the resulting tsunami even took lives far across the Pacific ocean. It’s not an experiment we would happily have chosen. But nature has carried it out without asking us; we may as well learn what we can from it.
When water hits hot magma and turns to steam, there’s an immense release of energy, especially if the magma is itself packed with compressed gasses. This is partly why some of the largest explosions in the last two hundred years have occurred when volcanic islands self-destructed; Krakatoa is the most famous. The latest estimate as of the time of writing is that the one in Tonga last week was overall perhaps only 1/20 times as powerful as Krakatoa, but its plume was enormous, and its shock waves were strong enough to be detected multiple times, in many places, as they traveled round and round the Earth.
The shock wave emanated from the explosion in all directions, moving outward as an ever expanding circle, as you can guess by pure reasoning but also as confirmed by satellite. After traveling 1/4 of the way around the Earth, the wave front reached a maximum extent — the same size and shape as the equator, though with a different orientation — and then shrank again, converging to a point in Africa exactly halfway around the Earth from the explosion’s location. (A nice visualization of this, and of what I’ll say next, can be found here.) Then the shockwave continued onward, again expanding to the Earth’s full extent, and then shrinking and converging on the very spot where it was created in the first place. And this process repeated, until the shock wave, gradually losing its energy, faded beyond the point of detectability.
This pattern of outward expansion, convergence to the opposite point, return-ward expansion, and convergence to the original point, means that the waves from the explosion passed every point on Earth multiple times, and did so first moving away from the explosion, then returning, then again moving away, and again returning, until finally they were too small to observe. That this pattern was seen everywhere, in countries widely spread around the globe, by both professional and civilian weather stations, gives some qualitative evidence that the Earth’s a smooth object with a rounded surface of some type. For example, here is the pattern of multiple waves crossing, returning, re-crossing and re-returning as measured by weather stations in China; we can see three wave passages clearly (the fourth is too dim to measure well). And here is a similar pattern in the Netherlands; though it’s only at one location, and only the main shock wave is detected, the shock is seen six times.
What’s nice is that for a sphere — and only for a sphere [see caveat below] — the equations I wrote earlier for a circular canal still hold, and importantly, they hold everywhere, and have to give the same circumnavigation time T and the same splash time ts. That’s because if you are on a sphere, motion away from the volcano (or indeed any point), in any direction, will take you on a circular path of length equal to the sphere’s circumference. On any other shape, this won’t be true.
[To be fair, I am making a couple of assumptions: for instance, that the volcano was located on a random, not special, point on the Earth. (For example, if the Earth’s surface was oblong instead of circular, then the two points at either end of the oblong are special.) To make a long story short, there are still loopholes to the argument I’m giving here, but they are only relevant if there are very special and unlikely coincidences. Additional volcanoes, would quickly close the loopholes.]
In particular, the equations I introduced earlier should hold in China, about 1/4 around the Earth from Tonga. And they should also hold in the Netherlands, much further from Tonga, in a quite different direction. If the Earth had an uneven shape, then the time to go round the Earth in the direction from Tonga to Beijing would be different from the time to go round it from Tonga to the Netherlands; you wouldn’t get the same T. And if the Earth had edges (as in the absurd flat-earth map), you would see reflection waves; you wouldn’t get the same T or the same ts, and the second big wave across China wouldn’t look like the original one retracing its steps (a fact which already gives qualitative evidence for a round Earth.)
Using publicly available data from anywhere in the world, including what I’ve shown you from China and from the Netherlands, we can check ourselves that the Earth’s a ball and measure its circumference. Let’s do it.
So as not to spoil the fun, I’m going to wait until after the weekend to post the results. You are all encouraged to gather your children together and to try to measure:
- T, the time it took for the waves to travel around the Earth; do this both with the data from the Netherlands and that from China; do you get similar answers?
- ts, the time when the eruption occurred; use both the data from the Netherlands and the data from China (make sure you’re using UTC time, so you don’t get confused by time zones). Do you get similar and roughly accurate answers? Is it close to the time reported in this article?
- v, the speed of the waves, which you can determine by watching how long it takes them to cross a part of China and comparing that time with the distance of that path; caution, make sure you trace a path perpendicular to the wave front.
- C = T v , the circumference of the Earth, equal to the time it took for the waves to circumnavigate the Earth times their speed. Can you get fairly close?
Caution: You’re not going to get exactly the precise scientifically-known answers, nor will your answers be perfectly consistent, because the data I’ve linked to was neither taken nor presented with scientific levels of precision. But you should be able to get within 10-20%, enough to convince you the Earth’s surface pretty darn close to a sphere. If you want more precision, I’m sure precision data is available (anybody have a good link?) [Also note that there are some extra waves seen in the China map, some of them reverberations from the original explosion, and some due to later, smaller explosions; they travel in the same directions as the original ones, showing they come from the same place. For our purpose here, just keep your focus on the biggest waves.]
The point is that we can learn the Earth is ball-shaped without ever stepping off the Earth, and in fact without even traveling; and we can even learn, from the timing, how big the Earth is. All it takes is a natural explosion, measurements from a few places, some logic, and simple algebra. The data is now publicly available, and every science teacher in the world ought to encourage their teenage students to do this exercise! Not only does it confirm we live on a sphere, it shows that one needs neither a photograph taken from outer space, nor a flight around the world, nor specialized map-making skills, to obtain that proof.
Three Dimensions: The Universe
Now what about the universe as whole? The Earth and Sun are carrying us along as they travel within a three-dimensional surface. What is its shape? How can we know? [There is also the question of the four-dimensional surface that makes up the space and time of the universe. I’m not addressing that here, that’s even more complex.]
A circle is a one-dimensional sphere; the surface of the Earth (not its interior) is a two-dimensional sphere. Could the universe be a three-dimensional sphere? We can’t stand outside it to find out. In fact it’s far from clear there is meaning to “outside” since, after all, it’s the universe, and might be everything there is. Nevertheless, we can imagine, at least, trying to do a similar experiment. If there were a huge supernova explosion, or a tremendous flare from a distant black hole as it ripped apart a star, maybe we would see the light arrive from one side of us, and then later see it arrive from the other side, and yet again from the first direction, and so on.
Back before we knew the huge scale of the universe and the tiny speed of light, that might have seemed plausible. We can’t hope to do anything like this, unfortunately. But it’s not because the question makes no sense. The natural Tonga volcano experiment worked thanks to the fact that it’s a small world (after all) and the speed of sound is relatively fast, so it all took less than a day or two. In the universe, it’s the reverse; it’s a big place and the speed of light is relatively slow. Our own galaxy, the Milky Way, is itself 100,000 light-years across [i.e. it’s so big that it takes 100,000 years for light, traveling at the fastest speed our universe allows, to cross it], so even if our galaxy were the entire cosmos, as was thought until the 1920s, it would take at least 100,000 years to do this experiment. And of course we now know the universe is immensely larger than our own galaxy; indeed the most recent map of galaxies extends out, for the brightest galaxies, as far as 10,000,000,000 light years. Hopeless.
Nevertheless, the possibility that the universe has an interesting shape, and though huge might be small enough that we could see some evidence of its shape, remains a topic of research. The light from events in the distant past might give us clues. While a blast wave isn’t something we’d be able to see from multiple perspectives, a long-lasting bright spot on the sky could potentially be seen reaching us from different paths around a complex universe. The fact that the universe has been expanding over the billions of years since the Hot Big Bang began complicates the thinking, but also provides opportunities.
To give insight into how this could be done is beyond the scope of this blog post, but if you’re curious about it, you might try this long-form article from Quanta Magazine (a highly recommended source for interesting articles.)
The Lesson for Humankind
The big lesson here: geometry can be learned from the inside. You don’t need to be outside an object to map it and learn its shape and size. That this is possible explains how mapmakers knew the shapes of continents long before satellites, and how one can determine that the universe is expanding while remaining within it (though the story of how scientists did this, without using the methods described in this post, is for another day.) And if the object is finite, so that no wave can travel forever without eventually returning to you, then it’s possible to infer its shape just by learning how waves travel and bounce around the object. That’s how the depth of the ocean’s deepest point was recently measured, as I described in my last post; and that’s how children (of all ages) should prove for themselves, using publicly available data from last weekend and simple algebra, that the Earth is indeed round.
2 Responses
As always, very intriguing article, you should write more, Prof.
So, did you just hypothesize the universe is closed, a 3-dimensional sphere, as it must be since we, matter, exist?
Here’s what I mean, the redshift only indicates the “local” size that we can measure with our technologies TODAY. So, yes, we measure it at ~ 13.5 billion years old, but that could only be a small sector of a bigger sphere, but we are limited by our sensors. Hopefully, James Webb Space Telescope and even more importantly, LISA, whenever they finish that, the launch date is predicted 2037 :(, will give us more data.
If it’s closed then a simple estimate would put the universe at ~ 78 billion years old, the complete sphere.
Why a closed sphere, must be the answer? Because, again simply, we exist, so there cannot be a beginning and an end, we just exist, the conservation of energy and the minimum limit, ZPE, clearly indicates that.
So, this hypothesis would imply that there’s an edge (surface) of the sphere spacetime continuum. Not necessarily, because if all the mass (energy) is contained within the sphere then no wave/particle can ever escape/reach beyond the gravitational pull of the spacetime continuum. So there is no edge, but the Black Holes could be where the spacetime continuum is pinched down to nothing, so these pinched regions are in fact the “edges” of the universe. Note that they could repair by Hawkings radiation and dissipate back up to ZPE level.
Here’s where this retired engineer turn cosmologist enthusiast puts his foot in his mouth, :), if this hypothesis is even close then the speed of light isn’t the “fastest velocity”, I would say that all of the energy (waves/particles) are permanently entangled with every Big Bang event. Yes, the universe must be cyclic. So, the fastest velocity is INFINITE.
PS: I’m just an old retired engineer, please be kind. 🙂
Very interesting, and especially useful to excite layman about what science, analysis and simple measurements can bring as understanding. An example that I will use. Thank you again.