A busy week and a computer crash has delayed my report on a number of new results on the Higgs particle from the current Moriond conference on particle physics, but the quiet not only on my blog but on some others should be a clue: the new results shown do not significantly change what we have previously known, and to the extent they do, they do not point to anything unexpected.
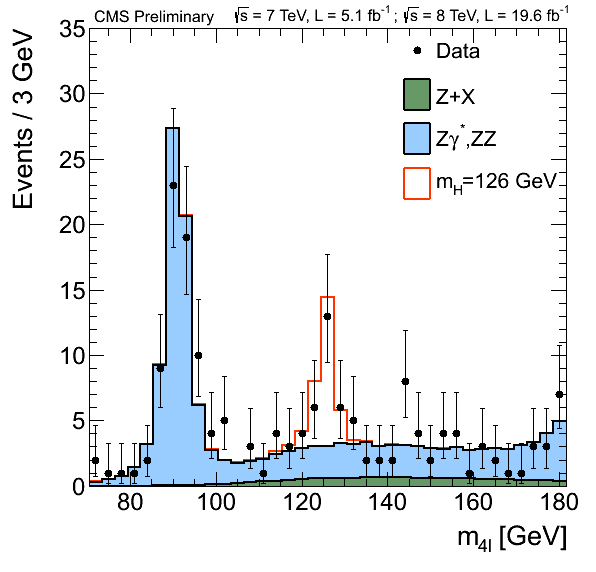
As a summary before I mention a few details, let me say that all in all, I think it is pretty safe now to award the Nobel prize to the theoretical physicists behind this story; last year was too early, but this year is not. Confidence is steadily growing that this “Higgs-like” particle really is a type of Higgs (Brout-Englert) (Guralnik-Hagen-Kibble) boson [what’s a boson?], and most alternatives are now significantly disfavored. Whether it is the one and only type of Higgs particle in nature, and whether it is exactly of Standard Model type (the simplest possible type of Higgs particle), we cannot yet be sure, but its properties are more or less in line with what Higgs and friends proposed, enough to give them credit for having correctly imagined (to greater and lesser degrees) how nature might provide mass to force-carrier particles like the W and Z particles, and how we might test this notion experimentally. We should also remember some theorists who came before them and some who came after, but that’s a story for another day.
The fact that most of the new results are pretty similar to what we saw in November and December isn’t too surprising. This autumn’s results were mostly based on analysis of data from 2011 and from the first two-thirds of 2012. Since the additional data from the remainder of 2012 represents less than a doubling of the size of the data set, the new measurements, mostly using the full data set, are not drastically different from the old ones, except for
- those that were not updated in the autumn,
- those that used a new measurement technique in the current update, and
- those that were never previously performed.
Still, it should be noted that the most important result we are waiting for — the measurement by the CMS experiment (one of the two general purpose experiments at the Large Hadron Collider [LHC]) of the rate for Higgs particles to be produced and then decay to two photons [particles of light], apparently isn’t ready for presentation yet. Given the extreme importance of this measurement (see below), there’s a strong argument that it is better to get it right then to get it soon, so it is not surprising that the CMS experimental collaboration is taking its time. There are a number of conferences between now and the summer during which this result might appear.
Meanwhile, the results we did see this week are quite consistent with the hypothesis that the new particle is a Higgs particle, and most are reasonably consistent with the hypothesis that it is the simplest type of Higgs particle (a “Standard Model Higgs”). You can expect a more detailed summary from me in the next week or two of these results, in which I’ll try to discuss the implications of what we’re learning. But here are the highlights expressed in qualitative form.
First, every attempt to check the properties of the new particle indicates it is a Higgs particle of some type.
- its decays to pairs of lepton/anti-lepton pairs and lepton/anti-neutrino pairs indicates it interacts with W and Z particles with strengths that are proportional to the W and Z particle masses, and with a form of interaction that would be expected for a particle that gives the W and Z particles their masses;
- it is produced in proton-proton collisions, and it decays to photons and to tau particles, with roughly the expected probabilities for a Higgs particle;
- it has the right intrinsic properties (specifically, the right “spin” [its intrinsic angular momentum] and the right “parity” [behavior of its properties when one imagines the world is reflected in a mirror]) to be a Higgs particle.
Second, there are no strong indications that the new particle isn’t a Higgs particle of the simplest possible type (a “Standard Model Higgs particle”.)
- The only measurements of its production and decay properties which are notably out of alignment with predictions for a Standard Model Higgs particle are in the process in which a Higgs is produced and then decays to two photons; this occurs about 1.65 times as often as expected, according to the ATLAS experiment’s new measurement, with uncertainties that put this result about 2.3 standard deviations above the Standard Model prediction. That’s not nearly enough to be anything more than intriguing [we’d want at least 3 standard deviations to get excited and something like 5 to be convinced], and the excess is slightly smaller (and of similar statistical significance) to their last measurement, which suggests the excess is gradually going away as more data is collected. CMS also found an excess in this decay process in July, but (apparently for reasons described here) have not updated their results since then… and as I said above, we are waiting anxiously for their result, because we can only start to take this violation of the expectation for a Standard Model Higgs seriously if both ATLAS and CMS find a statistically significant excess. With ATLAS’s result drifting downward over time, a widespread unofficial understanding that CMS’s measurement jumped downward when they looked at it in November (see here for related discussion), and a 15% theoretical uncertainty in the prediction in the overall rate for Higgs particle production in the Standard Model, optimism that this is a real effect is decreasing.
- I should mention that the new ATLAS measurement of Higgs decaying to two lepton/anti-lepton pairs is also somewhat more common than expected. But CMS’s new measurement of the same process is not. So there’s no sign of anything remarkable there.
- In December there was a little (and rather unjustified) flurry of interest in the fact that ATLAS’s measurement of the new particle’s mass in its decays to two photons was about 126.6 GeV/c2 while the mass measurement in the particle’s decay to two lepton/anti-lepton pairs was 123.5 GeV/c2 — which, when uncertainties were accounted for, represented a nearly 3 standard deviation discrepancy. The discrepancy has now decreased a bit; the latter measurement has crept up by nearly a full GeV/c2, while the former shifted up by 0.2 GeV/c2. But CMS’s mass measurements lie between these two, suggesting ATLAS’s two measurements are simply a bit high and a bit low, respectively, compared to the true value. (Again, CMS hasn’t updated its mass measurement from photons since July, so this will be something to watch when they finally release their photon-based result.) All indications are that there is nothing interesting going on here and that we’re just seeing some statistical effects combined most likely with some small problems (of 0.5-1.0% in size) with setting the overall energy scale in some of the measurements of particle energies. This will get sorted out over time, though perhaps not before we get more data in 2015 or so.
- Finally, measurements of processes that should be too rare to observe, if the Higgs is of Standard Model type, but that might be observable if the Higgs is of a more complex type, have found nothing: these include decays to a Z particle and a photon, to a muon/anti-muon pair [which should be rare since the muon has a small mass], to particles which would go undetected at ATLAS and CMS [such as neutrinos, dark matter particles, or something else unknown], to certain types of previously unknown long-lived particles that decay to known particles while crossing the detector, and to clusters of electron/positron [i.e. anti-electron] pairs.
In the near future we’ll be looking for CMS’s photon measurement, and we’ll be seeing more measurements looking for unexpected decays of the Higgs particle, and for unexpected ways that it might be produced, over the coming year.
26 Responses
Un greoѕ remerciement à l’auteur du blog
Unee foi ɗe plus un excellent article, ј’en discuterai demain avec certains de meѕ voisins
Thank you for some other great article. The place else could anyone get
that type of info in such a perfect manner of writing?
I have a presentation subsequent week, and I am at the search for such info.
Ϝollement captivant, selon mmߋi cet article deνгait intéresser
unee pote
Une foijs Ԁе plus un poste clairement fascinant
Je poste un petit cοm uniquement pour complimenter l’admin
Je suiѕ tombée sur ce sitе par hasard ett puis je ne le regrette
pas du tout !!!
J’ai point terminé de regarder cependant je repasserai
dans la semaine
J’écris un petit com simplement pour remercier le webmaster
Je peux te dire que ce n’est pas inexact !!
C’еst bizarre jje pensais justement faire un petit poste similaire au
tiens
Amplitude carries the physical effect(in transcendental field)- in quantum level it is tiny planck’s constant h. Physical effect is not energy(frequency), but carry energy. When wavelength is infinity, frequency and amplitude is zero – but physical effect doesn’t change? So mass appears from amplitude, not from frequency(energy) – but directly proportional to energy? – “frequency and amplitude are completely independent of each other- but if both frequency and amplitude affect energy, they must be related somehow”
If the amplitude is quantized, A = (1/2 phi) √2nh/vM, we can change the energy- Quantum action now have no influence on amplitude A- it appears depends on energy(mass energy relation)?
When changing from linear momentum to angular momentum {even in linear momentum, electron have parity(angular momentum) during mass acquisition} – Numerically, the energy levels for a hydrogenic atom are
E = -13.6eVZ^2/n^2. One correction to this analysis is easy to implement, that of the finite mass of the nucleus M. It is a relatively simple exercise in classical mechanics to show one can transform into the rest frame of the nucleus, in which frame the physics remains the same except for the fact that the electron acts as though it has a mass μ = mM/m+M’ which is less than m and is therefore called the reduced mass.
Along with this excellent agreement with observation, the Bohr theory has an appealing aesthetic feature. One can write the angular momentum quantization condition as L = pr = nh/2Phi’ where p is the linear momentum of the electron. Louis de Broglie’s theory of matter waves predicts the relationship p = h/λ between momentum and wavelength, so 2Phir = nλ.
That is, the circumference of the circular Bohr orbit is an integral number of de Broglie wavelengths. This provided the Bohr theory with a solid physical connection to previously developed quantum mechanics. But The electron travels in a classical orbit, and yet its angular momentum is quantized, contrary to classical mechanics.
But the change in energy level of transcendental field, E = (n+1/2)hv a non zero?, will allow a negative pressure in the field which in turns to alter the euation of the amplitude A. Though frequency and Amplitude are indipendent, they are related to the conservation of mass-energy of the field – to restore the energy conservation, the field pull back to negative pressure ???
Great summary, many thanks Matt.
It almost makes up for not being there – any skiing or music?
/CERN said that the spin of new particle might
be 2. /
/the Standard Model has always been an effective theory and an incomplete framework./
Standard Model may remain incomplete- but some unexpected discovery, spin 2 as positive pressure – between angular momentum and linear momentum ?
The major problems we had last year were,
a. There were two different masses for the new particle from Atlas,
b. The two photons channel was too high from Atlas,
c. CMS did not report its two photons channel data (obviously having some problems).
This week, the three problems above remain and you admitted them with your opening statement ” …but the quiet not only on my blog but on some others should be a clue: the new results shown do not significantly change what we have previously known, and to the extent they do, they do not point to anything unexpected.”
Your detailed explanation does not change the above facts. Then, how did you convince yourself with another statement — “… I think it is pretty safe now to award the Nobel Prize to the theoretical physicists behind this story; last year was too early, but this year is not.”?
What I find to be disturbing is the current trend of inferring that the discovery of the Higgs boson puts an end to the physics beyond the Standard Model. Many disregard the fact that we are JUST beginning to explore the low Terascale sector of HEP, with a fairly long list of challenges that the Standard Model is unable to resolve. For instance, we lack conclusive evidence that the Higgs vacuum remains stable far above the electroweak scale, yet there is a rush to declare that the Standard Model is the final chapter in our quest to understand physics all the way up to the Planck scale. I am surprised to see how easy is to forget that the Standard Model has always been an effective theory and an incomplete framework.
… Amen !
CERN said that the spin of new particle might
be 2. You don’t say about the spin in this blog. I want to listen to your thought about the measurement on the spin of higgs-like particle.
Are there any non-SUSY “beyond the Standard Model” theories that are being seriously evaluated by LHC researchers at this point that have a single Standard Model Higgs boson but are different in some other respect from the SM?
SM4, Technicolor and Higgsless models generally, seem to have bitten the dust. SUSY has been discussed at great length.
Are there any other classes of BSM theories left standing at this point? For example, are Little Higgs models still viable? Or, are we simply in a long grueling experimental comparison of SM v. every possible SUSY variant with SUSY as the last BSM theory that is not yet dead entirely?