© Matt Strassler [December 11, 2013]
It’s been a while since I gave you a status update on the search for supersymmetry, one of several speculative ideas for what might lie beyond the known particles and forces. Specifically, supersymmetry is one option (the most popular and most reviled, perhaps, but hardly the only one) for what might resolve the so-called “naturalness” puzzle, tightly related to the “hierarchy problem” — Why is gravity so vastly weaker than the other forces? Why is the Higgs particle‘s mass so small compared to the mass of the lightest possible black hole?
Back in mid-2011, when the Large Hadron Collider [LHC] was still very young, my colleague John Conway announced on his own blog (Cosmic Variance) that supersymmetry (specifically, supersymmetry as a solution to the naturalness problem, which I will call “natural supersymmetry” here) was essentially ruled out by the data from the ATLAS and CMS experiments at the LHC. Well, it took three seconds to see that statement was wrong — and the fact that people are still looking for signs of natural supersymmetry even now shows just how wrong it was. Why is it so hard to rule out natural supersymmetry? Because there are an enormous number of variations on this theme … a huge range of variants of supersymmetry that can address the naturalness puzzle. To rule them all out is a huge task! And it’s not one that a few months of LHC data could accomplish, by a long shot.
By mid-2012, with five times as much data, and many more ATLAS and CMS searches through their data, the situation was a little more complicated. At that point, it took a bit longer — a few hours — to check that natural supersymmetry wasn’t ruled out by ATLAS and CMS results. Two things complicated the investigation. First, there were results from a very important measurement made by the LHCb experiment (though the LHCb experimenters managed to confuse the public by erroneously claiming, twice, to have ruled out natural supersymmetry, or at least to have “put it in the hospital” — one of the more meaningless statements about physics that I’ve ever heard.) The other was the hints, soon to be a discovery, of a Higgs particle with a mass of 125-126 GeV/c²; this turns out to be light enough to be consistent with natural supersymmetry, but just a bit too heavy to be consistent with its simplest variants. Still, it was far too early to claim anything definitive about natural supersymmetry, or about anything else, at that time.
What about nowadays? Well, what took a few minutes in 2011 and a few hours in 2012 now takes six months of hard work. The 2012 data collected at ATLAS and CMS was far more extensive than the 2011 data, and the experimenters at ATLAS and CMS have had far more time to dig through it. After all their efforts, it’s now a major enterprise for anyone to understand, in a clear-headed way, which variants of supersymmetry are ruled out and which are not. But it can be done. We’re now, finally, in a position to start drawing important, though still incomplete, conclusions about natural variants of supersymmetry.
As I said, supersymmetry comes in a bewildering array of variants, each of which makes somewhat different predictions for the LHC experiments. Is it possible, using existing searches by ATLAS and CMS, to make definitive (or nearly definitive) statements about natural supersymmetry, not specific to any one sub-class of variants, but applying to all (or a very larger class) of natural supersymmetric theories? This is the question my colleagues (Jared Evans, Yevgeny Kats, and David Shih) and I addressed in a recent paper.
The answer, we showed, is “yes”. It’s a qualified “yes”, because there are a few small loopholes, but I do emphasize the word “small”… which is a tremendous improvement over mid-2012, when you could drive trucks through the loopholes. Here’s the argument:
First: we assume we are dealing with a variant of supersymmetry that is natural, which requires that Higgsinos (superpartner particles of the Higgs particles [recall supersymmetry requires 5 types of Higgs particles]) with mass below about 400 GeV/c² or so. [This is somewhat conservative; most natural supersymmetric variants would have them quite a bit lighter.]
Second, we assume the gluino (superpartner particle of the gluon, and very easy to produce) is accessible, in the following sense: it has a mass below 1400 GeV/c², low enough that the LHC would have produced some of these particles during the 2011-2012 data taking.
Then we note the following: if and when gluinos are produced in the LHC’s proton-proton collisions, then, in almost every natural supersymmetric model with an accessible gluino, one or more of the following phenomena commonly results:
- Missing Transverse Momentum: Striking signs that the collisions are producing both observable and undetectable particles, with the observed ones obviously recoiling against ones that were not observed.
- Top Quarks and Antiquarks: particles which are rather heavy (the top quark mass is 175 GeV/c²) and often decay to an electron or muon, an (undetected) neutrino, and a bottom quark [or their antiparticles.]
- Large Numbers of High-Energy Elementary Particles: (quarks, anti-quarks, gluons, leptons, anti-leptons, or photons); typical numbers are between 3 and 10 elementary particles per gluino, and thus 6 to 20 per proton-proton collision.
Finally, we point out that searches for each one of these experimental signatures have been carried out effectively, and with almost no assumptions, at both ATLAS and CMS. The searches for the first two signatures are so strong that it is virtually impossible for a natural supersymmetric model in which gluino decays produce top quarks and/or missing transverse momentum to have been missed, as long as the gluino mass is below 1000 GeV/c², and often as high as 1200 GeV/c². If instead gluino decays produce few top quarks and barely any missing transverse momentum, but still produce many quarks, anti-quarks and gluons, then the constraint on the gluino mass is a bit weaker, perhaps as low as 800 GeV/c² or so, but typically closer to 1000 GeV/c². [If leptons, anti-leptons or photons are abundantly produced too, then the constraints become stronger again.] We also pointed out ways that the searches for gluinos in this latter category might be improved for even better coverage.
This set of observations rules out the vast majority of natural supersymmetry variants with the gluino in the accessible mass range up to or near 1000 GeV/c². Only variants with heavier gluinos, or with gluino decays that avoid all three of the signatures mentioned above, or with unnaturally heavy Higgsinos, evade this statement. This result is summarized in the Figure below. Importantly, in contrast to many of the initial (i.e. 2011 era) searches for supersymmetry, which relied on the three key assumptions of what used to be the most popular versions of supersymmetry
- in any process, the number of superpartners can only change by an even number;
- the lightest superpartner [which is stable, by assumption 1] is a superpartner of a particle we know (and therefore, to avoid conflict with other data, an undetectable neutralino or sneutrino);
- the superpartners that are affected by the strong nuclear force are significantly heavier than the other superpartners of known particles,
our results apply even if you drop any or all of these assumptions. We also do not assume that supersymmetry is “minimal” — i.e., that the only new particles to be discovered are the superpartners of known ones (plus the extra Higgs particles that supersymmetry requires.)
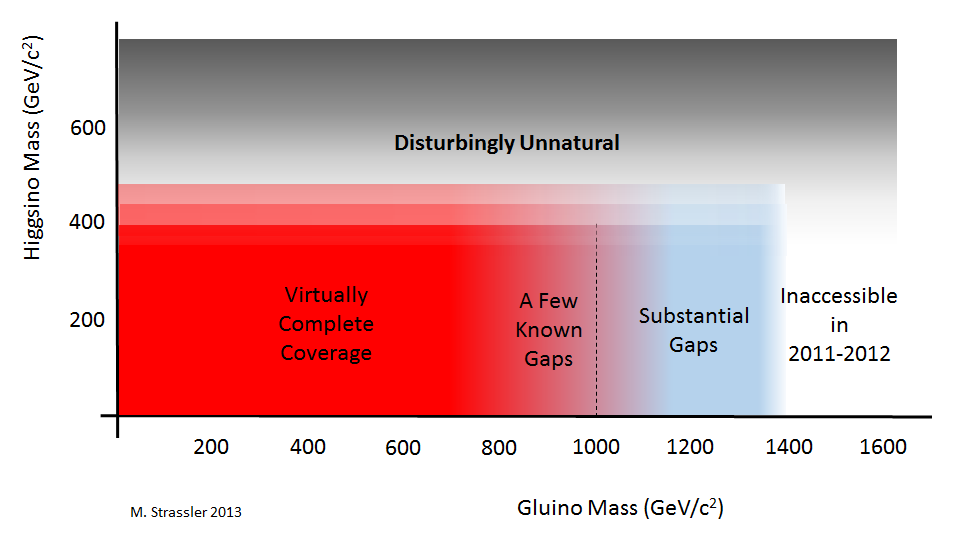
Where does this leave the search for natural supersymmetry? You might say it is about 3/4 done, roughly speaking. For natural supersymmetry variants that do not have a gluino that was accessible in 2011-2012, many searches have been carried out for other superpartner particles — but, as my colleagues Jared Evans and Yevgeny Kats have shown, one cannot say yet, in this case, that there is comprehensive coverage. For example, many searches for top squarks, the superpartner particles of top quarks, have been done, but each search has to make a certain assumption about how top squarks decay. There are clearly possibilities for top squark decay patterns for which no current search is yet effective. The same is true for Higgsinos and other similar superpartner particles.
Ruling out natural supersymmetry in a way that is nearly definitive, with very small loopholes of any sort, will not occur until after the LHC runs for a couple of years with the ~13 TeV proton-proton collisions that will start in 2015. But I think, by early 2017 if all goes well, we should be able to say that virtually all natural supersymmetry variants with a gluino mass below 1600-1800 GeV/c² are excluded (unless one is discovered, of course.) Limits on top squarks and on Higgsinos will also be much more powerful by then. And that will leave very little space for natural supersymmetry… little gaps will still remain, but the plausibility of the scenario will be very, very sharply reduced from where it is now, which is already greatly reduced from where it was before the LHC began running.
I should mention a couple of loopholes in our logic. The biggest loophole is that we assumed that there were no new long-lived particles, or other weird phenomena, in gluino decays. Such possibilities require an entirely different set of strategies, and are hard for theorists to study without knowing the details of how the detectors measure long-lived particles, a very complex subject indeed. For some types of long-lived particles, existing searches are very powerful; for other types, searches haven’t been attempted at all, so coverage is surely spotty. Second, and probably much less important, we assumed that the masses of the gluinos, Higgsinos and all other low-mass superpartner particles weren’t all squeezed together, within a few tens of GeV/c². Such scenarios require special treatment beyond what we covered; however it is not clear such a scenario is still possible within natural supersymmetry. Finally, one can in principle imagine gluino decays that are so complicated that they would confuse any existing search. Whether such decays could exist in practice requires more theoretical research. There are probably some other loopholes, but we believe they are rather small.
Another thing that’s important for non-experts to understand, and that is highlighted by our work, is that when the ATLAS or CMS experimenters say “we have done a search for X”, where X is some type of particle or phenomenon or speculative idea, that doesn’t mean the search is necessarily useless for Y, where Y is something altogether different from X. It’s important that the experimenters are looking for supersymmetry not only because supersymmetry might be there but also because the strategies they use may actually uncover something else. Conversely, sometimes searches for something with no connection to supersymmetry is secretly a useful way to look for certain variants of supersymmetry. [In short: even if one happens to believe strongly that X doesn’t exist, that doesn’t mean one should believe the search for X is a waste of time! One should not berate the experimenters for spending time “searching for supersymmetry” or “searching for extra dimensions” or whatever. This would be foolish, because the same searches are useful and needed for discovering or excluding many other things too.] For example: in our recent paper, we showed that a certain search for extra spatial dimensions (specifically, a search for microscopic, instantly evaporating black holes) is one of the most powerful studies for ruling out gluinos whose decays produce large numbers of elementary particles.
All in all, I’d like to think our results represent a step forward in understanding what ATLAS and CMS now tell us about particle physics, and in indicating how searches of future data ought to proceed. One possible lesson of our work is that in many situations, a small number of broad, comprehensive and powerful searches for generic phenomena can often trump a very large number of highly optimized but narrow searches for very specific phenomena. The latter leave far more holes, and, unless they specifically generate a discovery, are less useful than the former for drawing strong and general conclusions about nature.
57 Responses
Hi! Do you know if they make any plugins to assist with
Search Engine Optimization? I’m trying to get my blog to rank for some targeted
keywords but I’m not seeing very good results.
If you know of any please share. Appreciate it!
Welcome to “Solinea. Alveo’s first master planned, multi-tower residential development in Cebu City presents you with a brilliant living experience: the mellow glow of a home that is exactly the size you need, balanced with the invigorating vibrancy of everything under the sun.Solinea Tower 2will rise at an ultra-prime location right across Ayala Center Cebu, a privileged starting point for navigating the city in style. Via Cardinal Rosales and Luzon Avenue, joyride through 50 hectares of the best places to work, live, and chill, cityscapes unbound and energized by green urban spaces.
This page certainly has all of the information and facts I
wanted about this subject and didn’t know who to ask.
I think the admin of this site is actually working hard in favor of his website,
because here every data is quality based stuff.
Hmm iss anyone else encountering problems with the
images on this blog loading? I’m trying to figure out if its a problem on my end or iff it’s the blog.
Any suggestions would be greatly appreciated.
Check out the VTech Kidizoom Camera on sale at amazon. Fads and fashion can change quickly but you will not go far wrong by choosing a gift according to the boys age from this selection. a while, but now that we already have our first
4G LTE Android device on the Verizon Wireless network,
consumers want more.
Even in higher concentrations, it does not leave residue.
Ingredients:Method:Whisk together the mayonnaise and buttermilk in a bowl.
Have you every been to your favorite sub shop and wished that you knew the secret behind their tasty treats.
Most of the shampoo type products require you to shampoo
the hair, wait a few minutes and then lather up in the shower and rinse the hair.
Also soak the comb in the hot water and bleach for
about fifteen minutes and be able to dry the comb.
Remember proper dedication to this subject will prevent hours of
head lice treatment later.
Your style is very unique compared to other people I’ve read stuff from.
Many thanks for posting when you’ve got the opportunity,
Guess I’ll just book mark this blog.
“… the **MINIMAL** supersymmetric standard model is virtually ruled out by the data.” Would this ruling out also apply to the **Next-to-Minimal** supersymmetric standard model?
https://en.wikipedia.org/wiki/Next-to-Minimal_Supersymmetric_Standard_Model
What have physicists learned so far from LHC results concerning split supersymmetry?
https://en.wikipedia.org/wiki/Split_supersymmetry
No, the Next-to-Minimal supersymmetric Standard Model is not ruled out.
How sensitive are these results to the triggering strategies used in the 2011 and 2012 runs? If I recall correctly, in an earlier article you have mentioned the “parking” strategy of CMS and cited a similar strategy at ATLAS. Could you elaborate, if this is relevant for your results-or is it obvious that it isn’t?
Our results do not depend much on data “parking” or on any trigger subtleties. That’s because gluinos produce spectacular high-energy events with a very high rate relative to backgrounds, and so they are quite easy to trigger on. It’s precisely for this reason that we focused on them and were able to obtain general results.
However, if you wanted to make an airtight case not for gluinos but for top squarks or for Higgsinos, then data parking can make a big difference in closing some big loopholes. We should see results that require data parking within the next few months.
One other place where it may matter: if you have gluinos that decay furtively (because they decay to low-energy particles plus something invisible) or weirdly (to long-lived particles, for instance) then maybe, just maybe, you’d have a trigger issue. Our results do not apply to these two cases — the former, because it requires special signal simulation techniques that we weren’t using, for lack of computer time (but this case, for natural supersymmetry, is almost entirely ruled out already); the latter, because it requires detector techniques that no theorist can properly simulate. But again, the masses and energies and relative production rates involved are big enough that I don’t think data parking is important even here. It’s for lower masses and low signal-to-background that data parking can be really important… potentially. We’ll soon see if it was actually important.
How the experimentalists at CERN could avoid Supersymmetry vacua or extra dimensions or prediction of
incomplete multiplets of scalar and vector bosons ?
“There can’t be consistent spin-1 massless equations without a gauge invariance.”
“The electric field is to charge as gravitational acceleration is to mass.”
“Lorentz Invariance and Relativity are the same”
The relativity cannot explain the Lorentz invariance by its very definition, because it’s using it as a introductional postulate, i.e. the assumption, which is accepted without proof. It can be proven that , if one uses only the principle of relativity and one neglects the principle of light speed constancy, one can rederive Special Relativity.
If we admit the luminiferous Aether concept, the Lorentz invariance is the trivial consequence of the fact, the light is the only wave, which is used for observation and the relativity has nothing to do with such explanation. We can observe the Lorentz invariance even at the water surface, if we disable the using of the light both for time, both for space measurements.
“During my (Peter Higgs) first year as a lecturer I was in search of a worthwhile research programme. In the previous four years in London I had rather lost my way in particle physics and had become interested in quantum gravity.
Then in 1961 I read Nambu’s and Goldstone’s papers on models of symmetry breaking in particle physics based on an analogy with the theory of SUPERCONDUCTIVITY.
What I found very attractive was the concept of a spontaneously broken symmetry, one that is exact in the underlying dynamics but appears broken in the observed phenomena as a consequence of an asymmetric ground state (“vacuum” in quantum field theory).
Nambu had shown how spontaneous breaking of a chiral symmetry could generate the masses of spin-½ particles (We don’t have any known spin-1/2 massless particles in nature right now). But his model predicted massless spin-0 particles (pions?). Goldstone had argued that such massless particles would always be the result of excitations around the trough of the wine bottle potential.
“Goldstone Theorem,” appeared to put an end to Nambu’s programme.
Julian Schwinger had, as recently as 1962, written papers in which he demolished the folklore that it is gauge invariance alone that requires photons to be massless.
It became obvious that in this model the Goldstone massless mode became the longitudinal polarization of a massive spin-1 “photon,” just as Anderson had suggested.
Among the additional material was the remark, “It is worth noting that an essential feature of this type of theory is the prediction of incomplete multiplets of scalar and vector bosons.”
http://www.nobelprize.org/nobel_prizes/physics/laureates/2013/higgs-lecture.pdf
Prof. Strassler: Consider the following question concerning expert opinion on supersymmetry (SUSY): Suppose that P1, P2, P3, P4 are numbers such that P1 + P2 + P3 + P4 = 100 and P1 represents the % of experts who now think that SUSY is highly plausible, P2 = % who now think SUSY is moderately plausible, P3 = % who now think SUSY is somewhat plausible, and P4 = % who now think SUSY is implausible. What might be reasonable guesses for P1, P2, P3, P4?
I have no idea… and it depends who you define as an expert. There are people who claim to be experts who are not, and they often have skewed opinions.
It seems to me that “we’ve ruled out 75% of possible supersymmetric theories” is very different from “we’re 75% sure that supersymmetry is not real” (not that you have said or implied the latter). If you’re on board a remote ship at sea where there’s been a murder, and you’ve ruled out 75% of the passengers and crew as suspects, your belief that the suspect is somewhere on board will not have been reduced by 75% relative to the beginning of the investigation–you still probably have the same certainty that they’re there, somewhere. And in fact you already knew that there were N-2 non-murderers aboard, so identifying 75% of them doesn’t tell you anything new. Now, of course this is different! Because alternatives to supersymmetry are not as unlikely, a priori, as a murderer slipping away undetected from a ship. So, does ruling out 75% of potential supersymmetric theories cause your credence that some supersymmetric theory is correct change from x% to y%? If so, what are x and y, very roughly, for you personally Matt?
Correct; completing 75% of a search does not make you 75% more pessimistic, 25% as optimistic, or anything else. It just tells you that you’ve completed 75% of a search — that’s all. You keep looking until you’re done.
However, you might become more pessimistic if you had reason to think that the most likely place to find your target was within the regions you’d looked in already. I don’t think we have a strong enough reason to think that way.
I can’t put numbers on what I suspect or believe. Roughly speaking, I think it is surprising that supersymmetry, if it solves the naturalness puzzle, didn’t show up in the 1980s or 1990s. How much more surprised am I now compared to before LHC? Not that much. However, I *am* somewhat surprised that the Standard Model works so well. I would have thought we’d have more puzzles, if not new discoveries (possibly fake ones), by now.
Actually, the only thing I’m really surprised by is just how incredibly well the ATLAS and CMS experimental detectors work. They’re fantastic machines.
Does the Minimal Supersymmetric Standard Model predict a mass range for the superpartner of the magnetic monopole? https://en.wikipedia.org/wiki/Minimal_Supersymmetric_Standard_Model
If supersymmetry is discovered at the LHC, then the prediction is that all particles with mass far above 1 TeV/c^2 would have superpartners with masses that are quite close to their own. Assuming magnetic monopoles (if they exist) have very large masses, they and their superpartners would have very similar masses, differing by a small fraction. The heavier the monopole, the smaller the fraction.
By the way, the **MINIMAL** supersymmetric standard model is virtually ruled out by the data.
Is it possible for a particle to be it’s own superpartner, if it was heavy enough? (Just wondering, I don’t actually think there are any particles like that.)
Perhaps Astroparticles will give the answer on “where to search”
meaning that in such experiments, the sqrt(s) can be as high as >100TeV
therefore if statistics permit it , one could have a good hint on where to search and for what.
I really liked the “no waste of time” statement of yours.
Searching for irrelevant (at first sight) phenomena, might uncover SUSY or other interesting new physics and that’s one of the reasons why we should do it!!!
As a graduate student, I find boring the kind of searches : N – leptons , and M- jets, and MET — where N,M the number favoured by a specific scenario–
I really prefer more exotic stuff such as long lived particles – since we can do more creative experiments to trigger on them and find or exclude them.
Do you believe that this kind of search actually has a future??
Well, I think the Nleptons+Mjets(+MET) searches have their place; the really thorough searches of this type played a big role in the result I quoted. The important thing was to be thorough and to sweep the ground clean.
But searches for more exotic possibilities have to be done too. Not only do these searches have a future, they become more and more important when more straightforward search strategies turn up nothing. And if designed thoughtfully, a single search can cover a very wide range of models and signals. A particularly spectacular example of a powerful search for long-lived particles emerged recently from CMS: http://cds.cern.ch/record/1563591/files/EXO-12-038-pas.pdf . I still don’t think theorists have understood how completely this search wipes out models such as GMSB with displaced Z’s or Higgses, models with LSP stops or Higgsinos or gluinos that decay in flight via R-parity violation, etc.
I agree with you of course.
It’s just that from the my point of view, I wouldn’t choose such a search (N leptons, M jets , MET) for my phd, since I find it counter- creative experimentally.
See gluons on wiki, where you can read “as opposed to virtual ones found in ordinary hadrons”. And as Matt has said previously, a virtual particle is not a particle at all. So I don’t understand why people pursue SUSY and gluinos when we’ve never actually seen a real gluon.
Anybody?
a) do not learn physics from wikipedia.
b) http://cerncourier.com/cws/article/cern/39747 and http://profmattstrassler.com/articles-and-posts/particle-physics-basics/the-known-apparently-elementary-particles/jets-the-manifestation-of-quarks-and-gluons/
You didn’t answer my question. You gave me Ellis, the SUSY propagandist, and an essay which includes this: “It is a fascinating aspect of the physics of our world that when one of these particles is kicked out of the hadron that contains it, flying out with high motion-energy, it is never observed…” Yes, because it’s a virtual particle. It is not a particle at all. Which puts a new perspective on all those references to gluinos. I’m sorry Matt, but I think SUSY is really harming particle physics now. Every time somebody gives it some airtime people think sigh, another fairytale theory going nowhere, and they’re still peddling it.
Have you ever observed a tau lepton? Or a Z boson? or a W boson? or a neutrino? or a top quark? Have you ever observed an electron? Have you ever thought, for ten seconds, about what it means “to observe”?
I’ve thought a great deal about what it means to observe, I consider myself to be very empirical. And I care deeply for physics. I want what’s best for it. I’d just like to see more focus on robust “within the standard model” activities that are less open to criticism. IMHO there’s loads of great things to look into, things that really take you places.
No, I think you have not. For instance: the typical Z particle is MORE virtual than the gluons you claim aren’t real because they’re virtual. You haven’t thought about this carefully at all.
…and the top quark decays into a W boson and… You’ll forgive me if I don’t go into details, this is not the place for it. But I have thought about this carefully. And I’d like to make the point that there’s work required “within the standard model”, and that *promoting “beyond the standard model” instead is harming physics. For example, there are no magnetic monopoles in the standard model. It is better to spend a short time understanding why, than a long time searching for magnetic monopoles. Because one day somebody will explain why.
* I’m not accusing you of being some rah-rah propagandist for SUSY. You put in the caveats and you try to be impartial. But maybe you’re trying too hard, and putting up barriers to anything that would sway your impartiality. If so, please don’t. Be impartial about that too.
Well, if you think the gluon doesn’t exist, you’re foolish. That’s all there is too it. This has nothing to do with supersymmetry at all; it has to do with bread and butter physics of the Standard Model itself. The Standard Model without a real live gluon disagrees with data.
Foolish? When the gluons in a proton are virtual, and “a virtual particle is not a particle at all”? With respect Matt, I’m empirical. And this is everything to do with supersymmetry. Because you have got to understand the bread-and-butter Standard Model before going “Beyond the Standard Model”.
Remember your article What’s a proton anyway? Remember you said “there are zillions of gluons, antiquarks, and quarks in a proton”. Where do all those gluons go in low-energy proton-antiproton annihilation to gamma photons? When you can’t explain that satisfactorily, hopefully you will appreciate my point that there’s work required within the Standard Model.
Perhaps that tricky little question even has some bearing on your next blog entry. Think of the bag model, then think of the balloon analogy for the expanding universe. The pressure within a balloon is normally balanced by the tension in its skin. To make a balloon bigger, you can increase the pressure, or you can reduce the tension. Next time you’re talking to Sundrum, give him some bubblegum.
What you describe as your “Tricky Little Question” just shows you understand nothing about the subject. The gluons in the proton can annihilate with the gluons in the anti-proton just fine, thank you very much, just as the quarks annihilate with the antiquarks. Gluons are their own anti-particles, so the puzzle that you describe is no problem at all.
Good luck with your ideas! (balloons! hah!!)
The only content in your complaint is that indeed I have no choice, on a non-technical website, to take a few short-cuts. The crisp distinction between real particles and virtual ones is easy in some contexts, but not in a complex strongly-interacting system like a proton.
Specifically: if a particle travels without colliding with anything for a time T, then you can say it is “strictly virtual” and not a real particle only if its degree of virtuality is larger than h-bar / T. But a gluon inside a proton can travel only for a time 10^{-23} seconds or so before hitting the other side of a proton — and therefore its virtuality will always be of order 300 MeV or so. The “virtual” gluons you are referring to are actually not strictly virtual — they are as real as any particle could be inside a proton, in that their virtuality is as small as is possible inside a proton. In other words: in the sense that you are using the word virtual, and that I use it on this website, the quarks in the proton are just as virtual as the gluons!!!
And have you ever seen a quark? What makes you think they exist, if the reason you don’t believe gluons exist is because you’ve never seen one?
What a waste of my time… but hopefully this is useful to other readers.
So every single one of those zillions of gluons annihilates with another gluon? And yet the result is two photons?
Matt, with respect, none of your other readers are going to believe that. And again with respect, this sort of thing isn’t a waste of your time. Like I said you have got to understand the bread-and-butter Standard Model before going “Beyond the Standard Model”. And if you can’t explain something satisfactorily, that ought to tell you that here’s something you should thinking hard about. Please take this on board.
No reply necessary. You’ll forgive me if I avoid talking about quarks or other particles.
Try learning quantum field theory. At some point, if you actually want to understand physics, you actually have to learn it. If you (or any other readers) would rather disbelieve what I’m telling you, even though I’m considered one of the world’s experts on this subject and have been a scholar in this field for 25 years, and you’ve never worked through the equations yourself and taken the time to understand them, that’s your loss. Please don’t talk about any other particles; you’ve said nothing but nonsense since you opened your mouth about gluons.
[For readers who would actually like to understand: as I have tried to emphasize, protons are extremely complicated objects. Their interiors are very hard to characterize, and they are, indeed, full of quarks, anti-quarks, and gluons, with two more up quarks than up antiquarks and one more down quark than down antiquarks. BUT — quantum mechanics is a bit magical sometimes, and this is one of those places. A proton is a very special state, the LEAST ENERGETIC POSSIBLE ARRANGEMENT of those quarks, antiquarks and gluons. Such a notion makes No Sense in classical mechanics, but in quantum mechanics, it does make sense; and if it didn’t, atoms would collapse, instead of having electrons orbiting at a significant distance from its atomic nucleus. And therefore, despite Mr. Duffield’s assertion that this is obviously absurd, it is precisely true that when a proton meets an anti-proton, where that antiproton is the LEAST ENERGETIC POSSIBLE ARRANGEMENT of the corresponding anti-quarks, quarks and gluons (with two extra up antiquarks and one extra down antiquark) that it is possible for all the particles in the proton to annihilate with all of the particles in an antiproton, producing nothing but two photons in the end. Yes, Mr. Duffield, that’s exactly what happens.
BUT — Mr. Duffield, you’re not paying attention, as usual. YOU were the one who asked: what happens if a proton and antiproton turn into two photons. What you didn’t ask is: how LIKELY is that? In fact, for precisely the reason that it isn’t so easy for all of those zillions of gluons in the proton to annihilate with the zillions in the antiproton, it is in fact VERY UNLIKELY! What usually happens, instead, is that a proton and an antiproton annihilate into two or more pions, which are themselves made from quarks, antiquarks and gluons. In that situation, the zillions of gluons do NOT have to annihilate perfectly; most of them get incorporated into the pions that are the final state of the proton-antiproton annihilation.
So yes, it is very unlikely that all of the gluons annihilate; and yes, it is very unlikely that a proton and antiproton annihilate into two photons; but neither is impossible; and if a proton and anti-proton annihilate to form two photons, then YES, the gluons from the proton annihilate with the protons from the antiproton.
End of physics lesson, for those who actually want to understand something; and good luck to you elsewhere, Mr. Duffield, because I’ve had quite enough out of you and I do not want you around confusing my readers.]
Matt: I asked a reasonable question and your replies have not provided an adequate response. It’s not a matter of disbelieving what you’re telling us. It’s a matter of asking questions concerning the discrepancies within what you’re telling us. Saying things like try learning quantum field theory and saying I’m considered one of the world’s experts and then referring to magic (!) does not make up for an adequate response. The cross-section for annihilation to gamma photons cross section is not relevant. Because those pions are not the final state of the proton-antiproton annihilation. Those pions decay, don’t they? If you’d rather people like me weren’t around to point that out, then with respect, perhaps I’ve misjudged you.
“Foolish? When the gluons in a proton are virtual, and “a virtual particle is not a particle at all”? With respect Matt, I’m empirical. ”
No, John, you are not. You are a qualitative linguistic theorist. You take a statement phrased in common English for non-experts, and act as if your extrapolations from that statement are scientific fact. All the actual quantitative formulas and the empirical observations that support them be damned. Matt said “a virtual particle is not a particle at all” once therefore… whatever you think that statement implies is necessarily true, and true everywhere.
And then you take the balloon *analogy* which is only intended to explain how on an expanding surface everything else can be moving away from you without this implying you are at the “center”, and start talking about pressure and skin tension — because balloons have that, so it’s valid to talk about the universe like that, because the universe was once analogized to a balloon!
There’s not a scrap of empiricism there. Just a superficial understanding of non-technical language, *assumed* to be all the understanding necessary, enough so to claim that this understanding is “empirical” in nature.
Also, don’t speak for Matt’s other readers about what statements we’ll believe. I read Matt’s article on virtual particles, and his article on the structure of the proton, and have no problem reconciling the two, and have no problem understanding that within the complexity of the proton where strong forces are at play the distinction is muddy and you can’t actually point at 3 quarks and say “those are the real ones”.
Your belief that his responses are inadequate are yours alone.
Matt, I truly appreciate your patience, and your responses informative (less so than your articles or responses to better questions, so ideally you could focus your time on that but oh well).
duffieldjohn
first of all, what do you mean : actually SEEN a gluon?
We have MEASURED it! Had there not been for gluons we wouldn’t have mass:) (first of all) and second it’s been observed first in 1978 and re-measured numerous times ever since.
Gluon IS a real particle, as real as W,Z,γ,e,μ,τ,quarks etc
Is there an hierarchy (or fine tuning) problem within SM as a model (with no gravity, no black holes, etc. involved)?
Matt. :Allow me a comical question :
What would you say w.r.t Dr. David Chalmers 2013 claims of Panpsychism …ie photons , quarks etc are conscious !!!!
Matt. : Quo Vadis ???
In AWT the neutrinos are superpartners of photons, so that the SUSY is all around us. The existence of another super partners is speculative and broken with extradimensions.
That’s why nobody pays attention to AWT. You can prove neutrinos aren’t superpartners of photons on one line.
The supersymmetry operator Q changes a state consisting of a particle by itself into its superpartner particle:
Q | particle > = | superpartner >
Because Q carries no “charges” (no electric, weak isospin, or color charges) the particle and superpartner must have the same charges.
But the equation
Q | photon > = | neutrino >
is obviously wrong. The neutrino carries weak isospin charge; that’s why the Z particle interacts directly with neutrinos. The photon carries NO weak isospin charge; that’s why the Z particle and photon do not interact directly. That’s all you need to know; no neutrino can be a superpartner of the photon.
/* the particle and superpartner must have the same charges… */
But the superpartners of bosons are supposed to be a fermions and vice-versa. Bosons cannot carry the charge, so that the superpartners would be impossible.
Of course bosons can carry charges. W bosons have electric charge, isospin, etc. Gluons are bosons and have color and anti-color charge.
/*supersymmetry requires 5 types of Higgs particles*/
We already observed them http://i.imgur.com/jlleHw1.gif or not?
That’s a very funny picture; but indeed, no, because even if that graph weren’t a joke, it would show 5 *neutral* Higgs particles. But in [minimal] supersymmetry, three are neutral and two are electrically charged. There could be even more of them, but one can’t have fewer. All of the ones we haven’t seen could be quite heavy and hard to discover, unfortunately, so there’s no guarantee they will show up soon.
If there are multiple Higgs: are they each a ripple in their own independent Higgs field? Or are they different kinds of ripples in the same, single Higgs field?
Each is from its own field; but there can be little subtleties in the counting, which I tend to ignore when explaining things non-technically to the public. You can get some insight into this here: http://profmattstrassler.com/articles-and-posts/particle-physics-basics/the-known-apparently-elementary-particles/the-known-particles-if-the-higgs-field-were-zero/
Excellent summary of your paper. Two questions: (1) I am curious about your statement “The biggest loophole is that we assumed that there were no new long-lived particles, or other weird phenomena, in gluino decays.” . I thought long lived particles would be easier to detect, because you may not need theories for quick decays. What are these long lived particles anyway ( apart from neutralino)? Can you elaborate? (2) In the blog quantum diaries survivor, it is mentioned that CMS has ruled out quark-partners with charge 5/3 up to 800 Gev. I did not realize people were considering quarks with charges greater than 1. In SUSY models, do they enforce the Gell-Mann Nishijima?
Q=I(3)+1/2(B+S+…..)
a) The ATLAS and CMS experiments were not designed to find long-lived particles, and specialized techniques are needed to find them. Coverage is still spotty. There are many possibilities and it’s too long for a comment. Here’s an article http://profmattstrassler.com/articles-and-posts/lhcposts/searches-for-new-phenomena-as-of-92011/searches-aimed-at-supersymmetry/seeking-new-particles-with-long-lifetimes/ , and a recent talk: http://indico.cern.ch/getFile.py/access?contribId=38&sessionId=4&resId=0&materialId=slides&confId=255380
b) Yes, there are charge 4/3, 5/3, and even 8/3 particles that show up now and then in various speculative models. No, the Gell-Mann Nishijima relation doesn’t have to be enforced.
Do these results (such as the LHC neutralino searches) constrain the popular supersymmetric versions of WIMPs enough to impinge on the search for dark matter?
Probably not… you could still have a nearly standard supersymmetric WIMP as long as you’re willing to have the gluino heavy, although this typically requires something called a “Dirac” gluino, different from the minimal “Majorana” gluino, which introduces complications that I don’t recall precisely enough to explain right now.