We particle physicists live with the feeling that we are caged birds; we are safe and protected, yet we chafe to be free. Our cage is the Standard Model of particle physics, a set of remarkably simple equations (with remarkably complicated implications) that describe all the particles and forces that we know — excluding gravity, which we handle separately, and including the Higgs particle, which we haven’t understood or found yet (but are actively seeking). They allow us to predict all sorts of phenomena successfully, in advance, including the results of many dozens of experimental studies at the Tevatron and LHC.
We love these equations, and we hate them. They work so wonderfully well that they give us an incredible power — the power to predict and make sense of a tremendous range of phenomena. But they deny us power at the same time, because they leave a number of fascinating questions unexplained. Why does nature sport the elementary particles we know, and not other ones? Why do they have the masses that they do? Are the particles we know truly elementary, or are they themselves built from more elementary ingredients? Etc., etc. Only when we break free of the Standard Model will we have a hope of answering those questions. And that will only happen when the Standard Model makes a prediction that is demonstrably, spectacularly false.
Do we currently have a clear example of a failing prediction? There are always a few hints of failure running around, and most of them remain just hints. But we might have something better than a hint right now — there is reason for hope as well as considerable skepticism. The culprit? An asymmetry, to be described in a moment, in how heavy quarks and anti-quarks are produced in the collision of the lightweight up and down quarks and anti-quarks found in protons and anti-protons. The Standard Model predicts a very small asymmetry, but the experiments at the Tevatron, the CDF and DZero detectors, see a significantly more substantial one.
What asymmetry is this? It involves collisions of up quarks with up anti-quarks, or down quarks with down anti-quarks, that create a top quark and a top anti-quark.
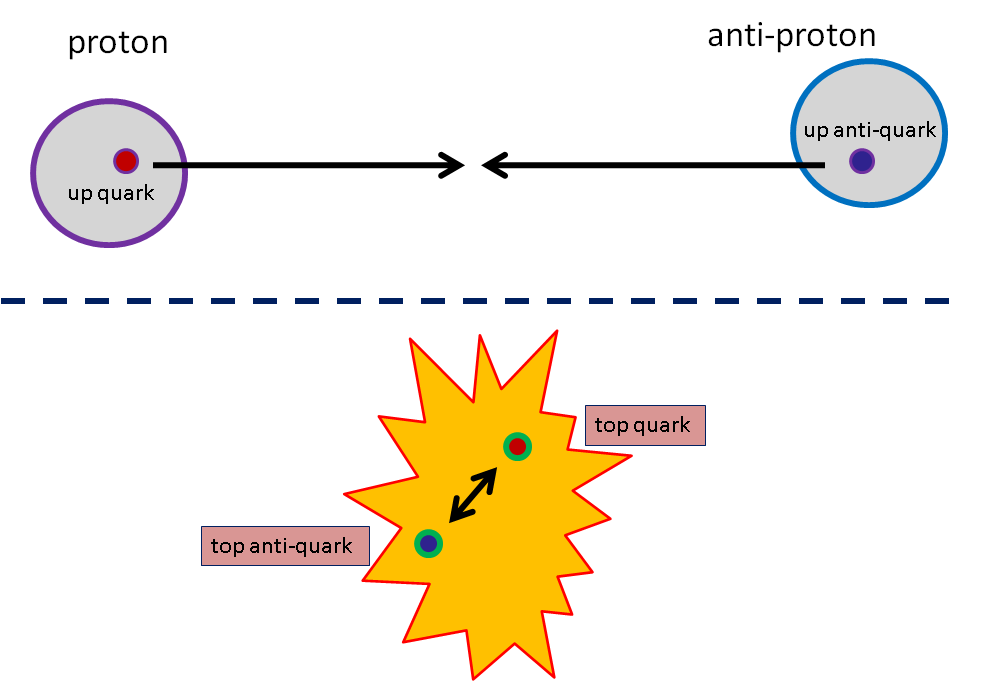
Unlike the LHC, the Tevatron is a proton-antiproton collider, as shown in Figure 1, and collisions that make a top quark and a top anti-quark can occur there. Sometimes people naively say a proton is made from three quarks (and an anti-proton from three anti-quarks), but this is wrong, as described in this article on the proton. In fact, there are innumerable quarks, anti-quarks and gluons in a proton, and also in an anti-proton. However, what is true about that naive statement is that the proton has three extra quarks — three more quarks than anti-quarks — and the anti-proton three extra anti-quarks. And one more thing is true, and very important, that I didn’t emphasize in my article on the proton, but is crucial here. The extra quarks in the proton are somewhat more likely to carry a significant fraction of the proton’s energy than are the quark and anti-quark pairs and gluons that are found throughout the proton.
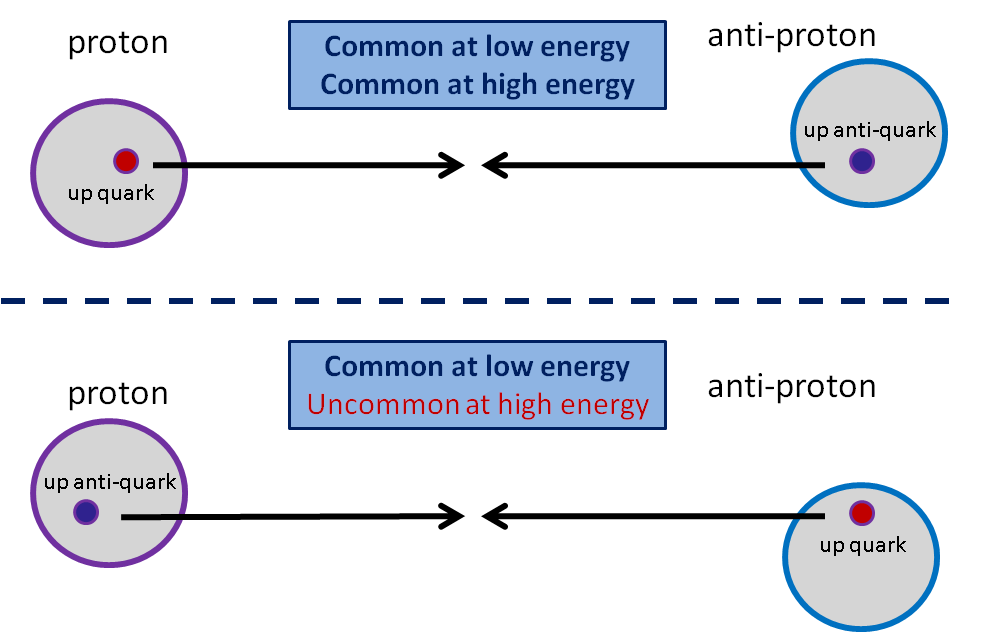
What that means is indicated in Figure 2: If I ask what is the most likely source of an exceptionally high-energy quark-antiquark collision at a proton-antiproton collider like the Tevatron, the answer is that an up or down quark from the proton probably hit an up or down anti-quark from the anti-proton. It can certainly happen that an anti-quark from the proton can hit a quark from the anti-proton, but the probability that such a quark-antiquark collision has very high energy is much, much lower.
Because the top quark is so heavy, it takes a lot of energy indeed — a substantial fraction, about 15% or more of the Tevatron collision energy — to make a top quark and a top anti-quark. And so we can infer that most of the time when a top quark and top anti-quark are produced, they were produced by a quark from the proton hitting an anti-quark from the anti-proton. Thus we know with high probability which direction the quark was moving prior to the collision! It was moving in the proton direction, almost certainly. Let’s call that the forward direction, and the anti-proton direction of motion the backward direction, as indicated in Figure 3. (Note that two colliding gluons can also make a top quark and top anti-quark, but this is rare at the Tevatron. It’s not at all rare at the LHC, though!)
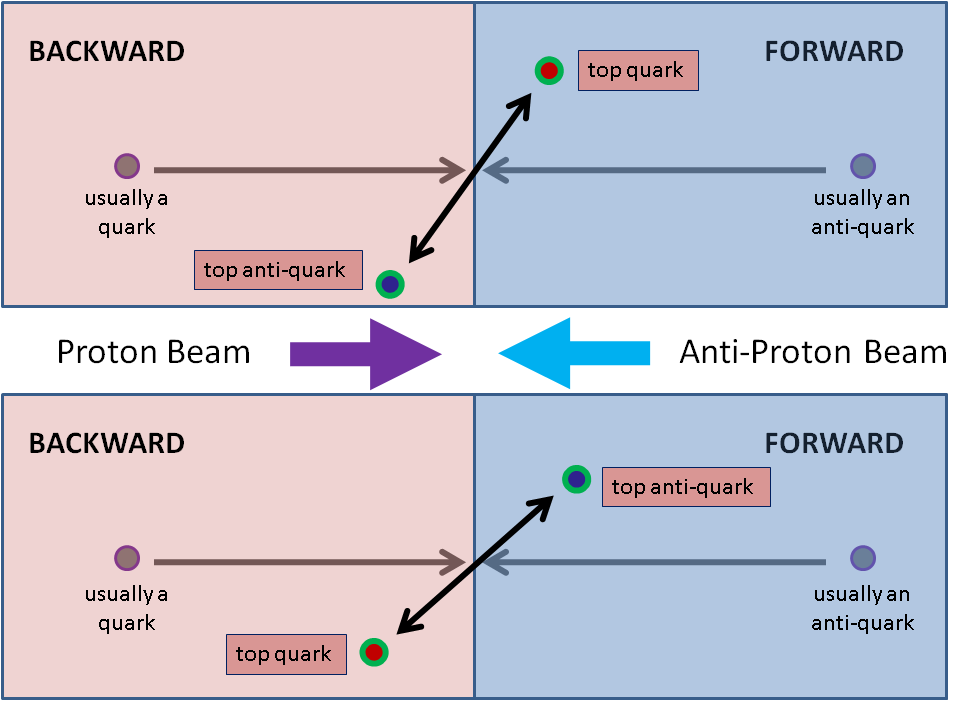
Now here’s the surprise. The Standard Model predicts that it is almost as likely that the top quark moves roughly in the forward direction (and the top anti-quark moves in the backward direction) after the collision as the other way around (with the top quark heading roughly backward and the top anti-quark heading roughly forward.) That is, in the Standard Model the upper and lower processes shown in Figure 3 are almost equally likely. Any preference for one over the other — the tendency of top quarks to go forward rather than backward, and top anti-quarks to go backward rather than forward — is described as an “asymmetry”. If the top quarks always went forward, that would be a 100% asymmetry. If the top quarks went as often backward as forward, there is a 0% asymmetry. The Standard Model predicts a 5 to 10% asymmetry, which is pretty small.
But CDF and DZero measure larger effects. How large depends exactly what you ask, and both experiments have made several related measurements, but numbers between 2 and 8 times larger than the Standard Model prediction have appeared in their articles. And the claimed uncertainties (i.e. error bars) on their measurements are small enough that the probability of these effects being purely statistical accidents are starting to become quite small.
Suppose that these are not statistical accidents. What are the possible causes?
- The theoretical calculation of the Standard Model process, like any theoretical calculation of its kind, is known to be incomplete. Perhaps the current calculation is not as accurate as people believe? Perhaps a better calculation would give a much larger effect?
- Some of the experimental measurements are tricky, requiring various types of manipulation and modeling of the data. Perhaps a subtle mistake was made that caused the Standard Model asymmetry expectation to be artificially inflated?
- Perhaps there are new particles or other phenomena which are causing the Standard Model to give the wrong answer?
We’d all like the answer to be option #3, to set us free of the Standard Model. But the most likely situation, as always, is something less exciting: options #1, #2, or a weird combination of accidents.
That said, no one has been able to make a convincing argument in favor of #1 and #2.
But meanwhile, theorists have been inventing many scenarios for #3, and finding that many of these scenarios predict other effects at the Tevatron or LHC that have recently been excluded by data at one collider or the other.
An example of a new scenario (largely excluded now, but easy to explain) is that a new light particle is being produced that introduces a new force that affects the top-left differently from the top-right. (As explained in and around Figures 2, 3 and 4 of this article, the top quark is a mixture of a top-left and a top-right that are mixed up with each other when the Higgs field becomes non-zero.) This, it turns out, could create a large forward-backward asymmetry for top quark-antiquark pairs.
But this force also would change other things, at the Tevatron and also the LHC, such as (a) increasing the rate for producing top quark-antiquark pairs as extremely high top-quark energy, and (b) increasing the rate for other processes, such as for production of pairs of up and down quarks. Neither effect is seen.
What can the LHC do for us in the context of the forward-backward asymmetry itself? Since the LHC is a proton-proton machine, not a proton-antiproton machine, the Tevatron measurements cannot be precisely repeated; the logic used earlier in Figures 1, 2 and 3 does not apply. But still, with more subtle tricks, it turns out one can dig out the same information — it just takes a lot of data to do it. The data collected over the next 18 months may be enough.
But the LHC should have other, faster ways of picking up new effects that impact top quarks. If there are new particles causing the excess asymmetries at the Tevatron, the LHC experiments may detect the effects of those particles in some other measurement. The fact that neither ATLAS nor CMS has seen any such effects already restricts the types of new particles that might be responsible for the discrepancies — assuming there really are discrepancies, and that we’re not just being fooled by a statistical fluke, a calculation error, or an experimental mis-measurement.
The situation remains very confusing. We all are hoping that this set of mysteries will be cleared up soon, so that we will know if our cage is finally broken, or if we should settle back in for another long night in the lovely prison of the Standard Model.
10 Responses
Do you think that ILC will be a more precise machine to investigate this problem? LHC, despite being the machine with highest center of mass energy, has serious problem of QCD background, which limits it’s precision for such sensitive measurements.
Another possibility is that undetected seismic activities (possibly even from Yellowstone area) disrupt the flow of energy on regular intervals, like a pulse. Energy seems to be fluid in its behavior, inasmuch, same energies always produce the same particles. What if particle is nothing more than a micro drop of energy that as soon as separation occurs, due to laws of QCD, it also assumes a charge. The charges of quark-antiquark and gluon-antigluon pairs is always in ratio of 1/3. The three main quarks absorb all the excessive energies and thus have larger mass. What keeps this consistency in the proton?
One question; do these collisions occur in nature ie., in supernova explosions?
P.S.
Yes, I know tevatron was closed in September 2011. Do you know what reason was given?
If energy created by the tevatron in these collisions is always at the same level, and protons have fixed number of particles then the cause of asymmetry could be either one of the following; tevatron cables are damaged and need replacing, EM energy is not constant even though it is expected to be, protons do not have a fixed number of quark-antiquark and gluon- antigluon pairs. May be nature is not as precise as the mathematical equation requires them to be. In the last case, particles must have ability to compensate for these naturally occurring inconsistencies.
This is probably a dumb question, but suppose the top quarks and top antiquarks were themselves composite particles, so the scattering cross section form factor was dependent on the momentum transfer. Then wouldn’t this favor forward scattering ( 90 degrees in cm)?
should read “forward scattering (under 90 degrees in cm)”
Not a dumb question. But in fact the way that top quarks are produced would not give you the effect you want; your intuition is off because the production of a top quark occurs (dominantly) through a single virtual gluon, which eliminates the forward-versus-backward distinction. You need another source. Moreover, if compositeness gave such a large effect as is being observed, it would be observable in other measurements; but (as of July 2012 — there’s much more LHC data now than there was when this article was first written) no effect is seen.
In the paragraph around Figure 3, you say: “And so we can infer that most of the time when a top quark and top anti-quark are produced, they were produced by a quark from the proton hitting an anti-quark from the proton.” Shouldn’t the end of this sentence be “…an anti-quark from the anti-proton”?
yes, thanks!