Matt Strassler [March 7th, 2012]
One of the important things to know about jets [sprays of hadrons that are the manifestation of high-energy quarks, anti-quarks and gluons] is that most quarks, anti-quarks and gluons turn into jets that look pretty much the same. But one of the other important things to know is that there are exceptions to that truism. Jets from bottom quarks or anti-quarks, and to a much lesser extent from charm quarks and anti-quarks, can be distinguished from other jets. Let’s see how it’s done. It’s all because of a fortunate accident.
Most particles decay, but it is very important to look at what their average lifetimes are, because that determines how far a fast one (traveling at a substantial fraction of the speed of light) can go before it falls apart. The various quarks have very different lifetimes.
- up and down quarks can survive basically forever (with caveats that have to do with the details of nuclear physics; let’s ignore that for now)
- strange quarks live long enough that they can travel large distances — meters (or feet, if you prefer) — before decaying
- top quarks live such a short time that they can’t even cross a proton before falling apart!
- bottom quarks, and to some extent charm quarks, have lifetimes in a “sweet spot”; they typically travel a fraction of a millimeter (i.e. they travel something like 1/100 of an inch) before they decay.
The distance that bottom quarks typically can travel, and occasionally charm quarks too, turns out to be an ideal distance for detection by the LHC detectors ATLAS and CMS, and the Tevatron detectors CDF and DZero, and many other experiments too. Let’s see how this works.
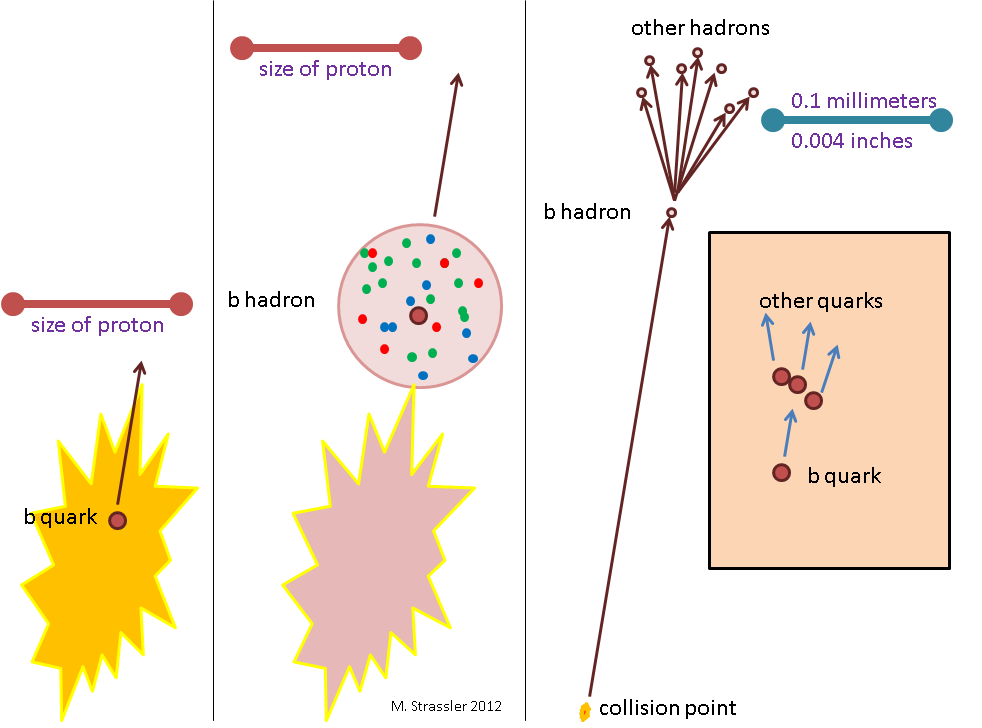
In Figure 1 is shown the progression of what happens to a bottom quark. At left is shown a proton-proton or proton-antiproton collision in yellow, and somewhere in that collision a high-energy bottom quark is produced. We’ll ignore what happens to all the other quarks in the collision, and just follow this quark along its way.
In the middle of Figure 1 we discover that while traveling out of the collision region, the bottom quark has surrounded itself (as a result of strong nuclear forces — see the discussion of jets to understand a bit of why this happens) with an anti-quark (perhaps an up, down or strange anti-quark) and lots of other quarks and anti-quarks and gluons. We call this messy object that surrounds the bottom quark a “hadron” in general, and more specifically a “bottom hadron.” (It might be a “B meson” or a “B baryon”; the latter is like a proton or neutron but with one down quark replaced with a bottom quark.) As in the previous panel in the Figure, there are actually lots of other hadrons coming out from the collision point, but we’re ignoring them to focus on this one.
[Hadrons are complicated; there’s no way around it. The proton is a classic example, and you can learn about its complications here and here and here.]
On the right of Figure 1, we step back — waaaaaaaaaaaaaaaaaaaaaay back. Notice the change in scale!!! What was previously the size of the proton is now a tenth of a millimeter. The hadron is now so tiny that if I drew it to scale you wouldn’t be able to see it at all, so I’ve drawn it much, much, much bigger than it really is. But I’ve indicated how it has rushed away from the collision point (also drawn too large, so you can see it). And at a distance of a few tenths of a millimeter, the bottom hadron falls apart, into half a dozen to a dozen other hadrons, none of which contain a bottom quark.
Why did this happen? That is shown within the inset at right of Figure 1. The reason the bottom hadron fell apart into other hadrons is that the bottom quark itself fell apart into a two quarks and an anti-quark — for instance a charm quark, a down quark and an up anti-quark. The reason so many hadrons are produced in the bottom-hadron’s decay is partly related to why jets form; the strong nuclear force is responsible for many complicated effects, and this is yet another one! But there’s more to this story, which gets rather long; let’s skip it for now.
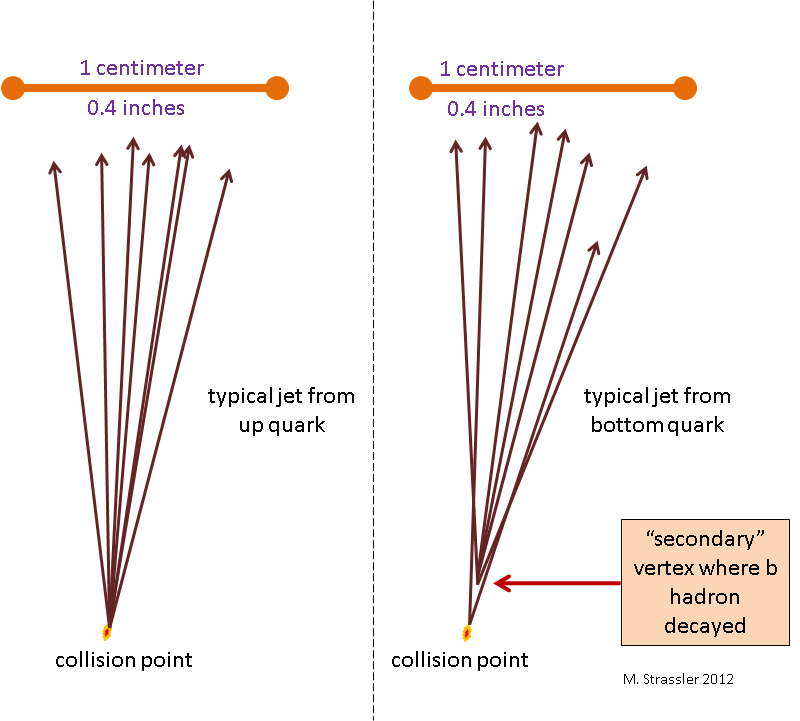
Finally, in Figure 2 we step back even further, so you can see the trajectories of all the hadrons in a jet. On the left is shown the trajectories of the hadrons coming out from a typical jet, let’s say, one from an up quark. You notice all the hadrons have trajectories that seem to come from the collision point where the two protons, or the proton and anti-proton, collided in the first place. But on the right is shown the trajectories of the hadrons from a jet that was initiated by the production of a bottom quark (or anti-quark). A significant number of the hadrons in this jet came not from the collision point but from a point further out; the point where their trajectories cross, called a “secondary vertex”, is the location where the bottom hadron decayed, as shown on the right of Figure 1.
Now how do you “b-tag” a jet — i.e., identify it as likely to have come from a bottom quark or anti-quark? You measure the trajectories of the hadrons very precisely, using the tracking systems that ATLAS, CMS, CDF and DZero (and many other experiments) all have. Now, that’s not quite a simple as it sounds. First, you can’t measure anything about the trajectories until the hadrons have traveled a few centimeters, so you don’t see the bottom hadron directly; it decays long before it exits the metal pipe in which the proton and anti-proton beams travel. Also, with modern trackers, you only “catch a glimpse” of the hadron every few centimeters or so, as it travels outward; knowledge of the track isn’t continuous. But still, you can reconstruct the track for each hadron that makes its way through the tracker, in a sort of “connect the dots” approach. And by making very precise measurements of all the tracks, you can detect that some of the tracks in the jet did not come from the original collision point, i.e. that the jet looks more like the one on the right of Figure 2 than the one on the left.
By the way, you can determine the original collision point by tracking other hadrons coming from other jets (not shown) and from the debris from the proton-proton collision (also not shown).
So that’s it. That’s how “b-tagging” is done. In principle it is not really very sophisticated. You simply
- measure all the tracks in a jet as accurately as you can,
- extrapolate them back toward the original collision point, and
- ask whether any of the extrapolated tracks miss the collision point.
If they do, that’s a sign that there was a hadron in that jet that traveled a good fraction of a millimeter, and there’s a good chance (not a guarantee) that this was from a bottom quark or anti-quark.
Of course then you make b-tagging much more sophisticated by playing all sorts of additional subtle and clever tricks. That gets very technical, so suffice it to say that by the time the experimentalists have managed to get the most efficient b-tagging possible, it’s far beyond what they can explain to me, much less me pass on to you. We non-experts basically have to trust that they know what they’re doing… and the successes of the Tevatron and the LHC where b quarks are concerned suggest that they do.
5 Responses
Don’t worry about the ambiguous future, just work hard for the sake of clarity
Thank you for your clear and nice explanation 🙂
Thank you for the great explanation – I agree with Aman it is a great introduction 🙂
Thanks for such a clear description! 🙂 Even though I am a physics masters student (doing my thesis on jets), resources like these prove to be great starting points.