Why is it a good idea to look for new physics at the Large Hadron Collider (LHC) in collision events that produce three or more charged leptons or anti-leptons? The basic principle is simple. Leptons are rarely produced at a proton-proton collider by effects of the known particles and forces (the “Standard Model” of particle physics). Two leptons (or anti-leptons) are still more rare; to make three at once is very rare indeed. And a variety of speculative ideas for new physics that might be observable at the LHC can significantly increase the rate for producing three leptons and/or anti-leptons in collisions where the total detected energy — from the leptons/anti-leptons and/or other particles (neutrinos, quarks, anti-quarks, gluons, or new invisible particles) — is high. So without being too specific about what new physics we’re looking for, we can go check to see whether the rate for multi-lepton production is consistent with the small rate predicted by the Standard Model’s equations.
Topics to be covered here:
- Why are multi-lepton events so rare?
- Fake isolated leptons: why studying rare events can be very hard
- What kind of new physics can produce multi-lepton events?
Why are multi-lepton events so rare?
At a proton-proton collider, quarks, anti-quarks and gluons are made with ease. There are quarks, anti-quarks and gluons inside the proton ready to have collisions; and when such particles collide, the most likely thing to happen is that they will scatter off each other like two billiard balls, causing them to fly out sideways to the beam. Such particles then are transformed into sprays of hadrons, called “jets”. And the next most likely thing to happen when quarks or anti-quarks or gluons collide with one another is that they produce new quarks, anti-quarks or gluons in the collision. These, too, form jets. So jets are commonplace at a proton-proton collider.
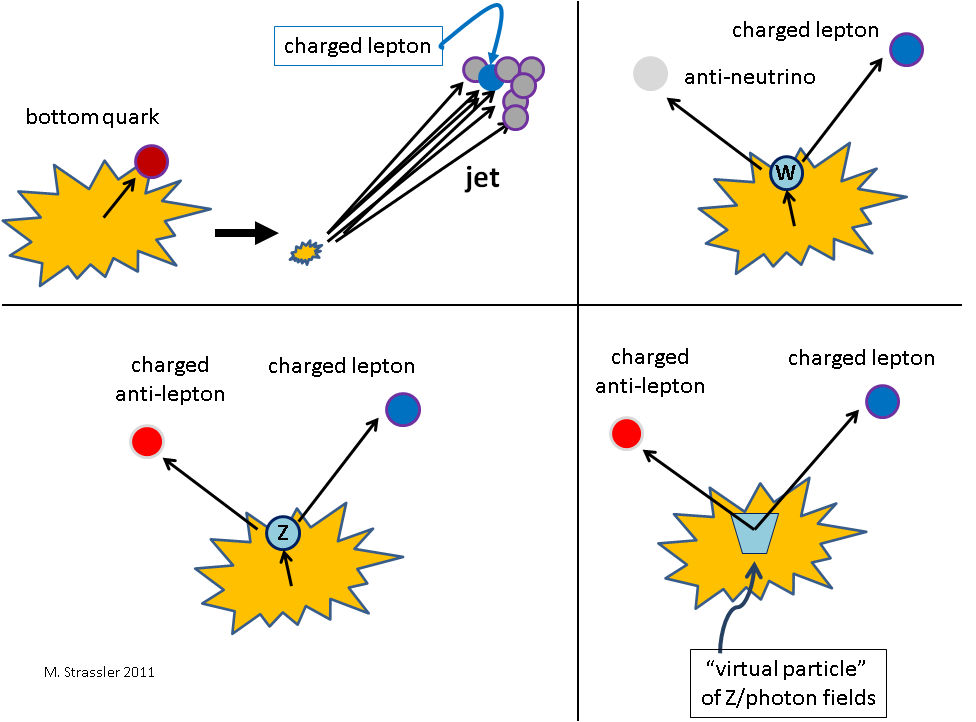
It is much more rare for leptons and anti-leptons to be produced. (Throughout the following I’ll often say leptons but mean both leptons and anti-leptons; this should be clear from context.) The most common ways to produce them (see Figure 1) are the following:
- 1 lepton, in about 20% of the decays of a bottom or charm quark, or even 2 for bottom quarks. But these types of leptons are usually inside the jet associated to the bottom or charm quark; they are not “isolated”.
- 1 isolated lepton, from 30% of the decays of a W particle (or also a W “virtual particle” — not a particle at all, but a more general disturbance in the W field.)
- 2 isolated leptons (specifically a lepton and anti-lepton of the same “flavor” — electron, muon or tau), from 9% of the decays of a Z particle.
- 2 isolated leptons (specifically a lepton and anti-lepton of the same “flavor”) from “Z or photon virtual particles” (a more general disturbances in the Z field or photon [electromagnetic] field; actually these are not really distinct because these disturbances mix with one another.)
- A top quark decays to a W+ particle and a bottom quark; the W can produce an isolated anti-lepton 30% of the time, while the bottom quark can produce 1 or 2 (generally non-isolated) leptons/anti-leptons.
Bottom and charm quarks are common, so leptons are not that uncommon. But isolated leptons are only produced by W and Z particles (rare because they do not feel the strong nuclear force and must be produced through the weak nuclear force) and top quarks (rare because they are quite heavy and relatively hard to produce).
- An isolated lepton from a W particle is made once every 10,000,000 collisions or so.
- An isolated lepton and anti-lepton of the same flavor are made from a Z particle once in every 100,000,000 collisions.
- An isolated lepton and anti-lepton of the same flavor that do not come from a Z particle (and therefore have energies that do not add up to the Z mass-energy [almost true; see here]) requires a Z or photon virtual particle (roughly once in every 1,000,000,000 collisions).
- An isolated lepton and anti-lepton of not necessarily the same flavor, from a top quark and a top anti-quark (which are most often produced together, see Figure 2) are made once every 10,000,000,000 collisions; these collisions, unlike those with Z or photon virtual particles, tend to have jets from a bottom quark and a bottom anti-quark, and signs of invisible particles (“missing `energy’ ”) from a neutrino and anti-neutrino.
- To get three isolated leptons and/or anti-leptons requires a W and a Z particle be produced together (which is rare, since this requires a double use of the weak nuclear force), and that they both decay as required (which happens less than 3% of the time); this occurs once every 100,000,000,000 collisions
- Three isolated leptons and/or anti-leptons, where no lepton/anti-lepton pair could have come from a Z particle (therefore requiring they come either from a virtual photon or Z [in which case they have the same flavor] or from a top quark/anti-quark pair [in which case they may not]) happens once every few 1,000,000,000,000 collisions.
All of these numbers are very rough, for many reasons; they are just intended to give you the basic sense of things.
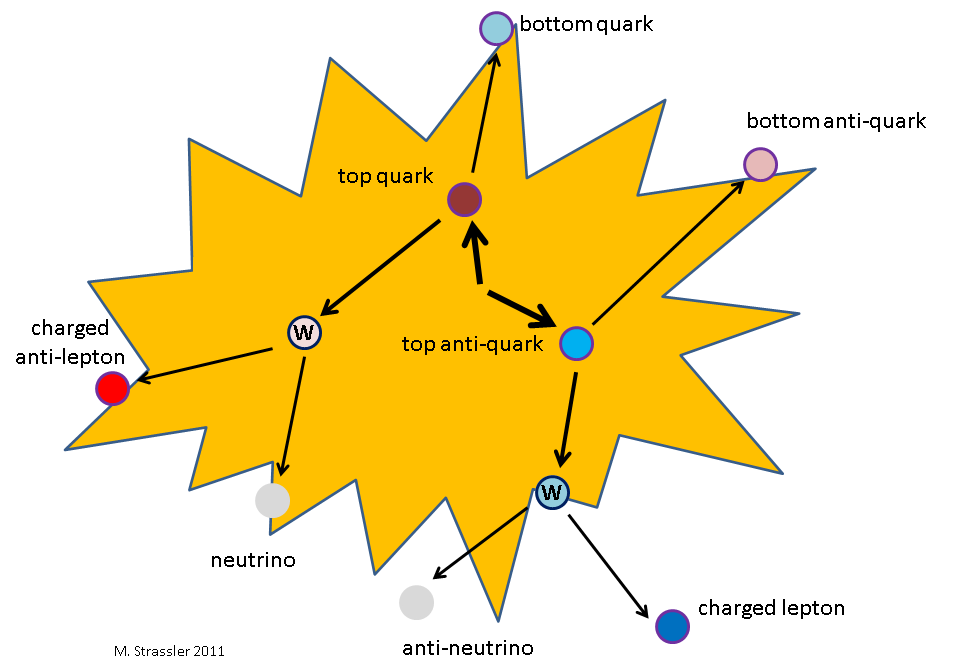
For scale, as of October 2011, each LHC experiment has seen about 500,000,000,000,000 collisions, and analyzed less than half of that data. And since the probability of detecting all three isolated leptons in a single collision that produces them is less than 25 percent, the number of events recorded of this last type so far is less than 100.
Fake isolated leptons: why studying rare events can be very hard
Moreover, many of the events of this type that are observed by an LHC detector are not what they seem. There are sources of extra leptons that have to be accounted for, stemming from the fact that detectors are not perfect, and from physical effects that I haven’t yet mentioned. These involve rare accidents, but since the sources of isolated multi-lepton events are rare too, they can’t be ignored.
- I emphasized that there are many bottom quarks and charm quarks that produce leptons in their decays. These tend to be non-isolated, and they also tend to carry rather low energy. But occasionally they are isolated, just by chance. As you try to search for multi-lepton events with lower and lower energy, the more of these accidentally-isolated leptons you will find, and the more often one of these will be combined with an event with two isolated leptons from other sources to make a three-lepton event. For instance, this can easily happen in a collision where a top quark and top antiquark are produced, as in Figure 2; the W+ and W- particles produced in the top and anti-top decays can produce a lepton and anti-lepton, and either of the bottom quark or bottom antiquark can accidentally produce an isolated lepton.
- Sometimes a jet from a quark, antiquark or gluon will look like an electron. This can only happen if the jet is very unusual and a couple of accidents happen, but there are so many jets at the LHC, and electrons are so rare, that this possibility cannot be ignored.
- Fake taus (and anti-taus) are quite common, because taus (unlike electrons and muons) will decay before passing through a detector, and a jet will occasionally look like the decay of a tau lepton. This is described in more detail here. Any search involving taus will have larger backgrounds than those that don’t, and one cannot study taus at as low an energy as one can manage for electrons or muons.
- Another important background stems from an effect of virtual photons that produce a low-energy lepton and a low-energy anti-lepton, one of which has so low energy that it is undetected or, if detected, not firmly identifiable as a lepton. This particular background has been emphasized especially by my theoretical and experimental colleagues at Rutgers in a recent joint paper, and it’s so important, interesting, difficult to calculate and underappreciated that it deserves its own article.
There are other potential sources of undesirable leptons or fake leptons too. The rates for all of these rare processes have to be accounted for; they cannot be calculated, so they must be measured in the data! It is absolutely essential this be done right, since for many questions these rare fakes actually form the largest source of background, rather than the more calculable backgrounds I described in the previous section. This is the great challenge in looking for rare physical processes; one has to worry about very rare accidents which can make an unrelated object resemble one’s quarry. A great deal of cleverness on the part of the experimenters is required; carrying this out properly is quite a tour de force!
What kind of new physics can produce multi-lepton events?
Many types of new physics can give multi-lepton events. One way nature could do this (Figure 3) is if there is a new particle that can produce a W or Z particle in its decays, or even just produce an appropriate disturbance in the W or Z field (called a “virtual particle”, but not really a particle at all.) If the new particles can be produced in pairs (i.e., the particle along with its antiparticle, typically), then it is common for the event in question to include both a W and a Z particle, or virtual particles from both W and Z fields. This can lead to three leptons.
In some theories, the presence of lepton-like partner particles (such as lepton superpartners in supersymmetry, also called “sleptons”) can participate, replacing the W and Z. Since they decay to leptons or neutrinos all of the time, they can often enhance the number of multi-lepton events compared to events with a W and Z, only a few percent of which produce three leptons.
Another possibility (Figure 3) is a new particle that can produce a top quark and top anti-quark in its decay. Then if that particle is produced in pairs, two top quarks and two top anti-quarks will result, leading to four W particles (one from each top quark or anti-quark.) While four leptons are possible, three leptons are much more likely (and even in four-lepton events it is common that one goes undetected.)
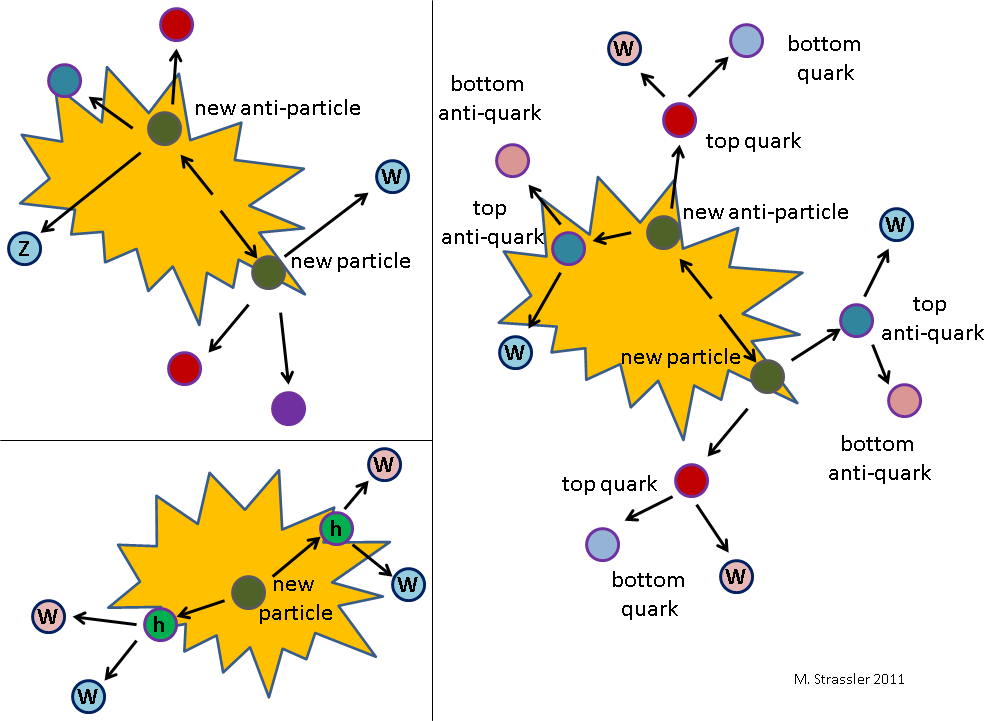
A new particle that is produced alone might also be able do the job. One possibility is a new particle that can directly decay to a W and a Z. Another possibility, if the Higgs were heavy enough to often decay to a W+ and W– particle, would be a new particle that could decay to two Higgs particles; if the new particle were produced, the chain of decays (Figure 3) could give four W’s and therefore up to four leptons in an event (though this particular possibility is now rather constrained by Higgs-particle searches.)
There are many other possibilities. However, the most famous motivation for the multi-lepton search comes from supersymmetry. You can read about why many variants of supersymmetry can produce multi-lepton events here. However, as I’ve just emphasized, supersymmetry is by no means the only or even the most likely new-physics source of multi-lepton events. If an excess of events really does emerge at the LHC, we’ll need a lot more information than just the presence of an excess to diagnose what is causing the events.
6 Responses
thanks
nice post
see more
http://profmattstrassler.com
These are really a superb showcases here I am glad to have a review of it and feel to roll on its steps for my benefit always
University in Haryana
good post shared here.
sarwar