[Note Added: Thanks to some great comments I’ve received, I’m continuing to add clarifying remarks to this post. You’ll find them in green.]
It’s been a couple of months since the `photo’ (a false-color image created to show the intensity of radio waves, not visible light) of the black hole at the center of the galaxy M87, taken by the Event Horizon Telescope (EHT) collaboration, was made public. Before it was shown, I wrote an introductory post explaining what the ‘photo’ is and isn’t. There I cautioned readers that I thought it might be difficult to interpret the image, and controversies about it might erupt.
So far, the claim that the image shows the vicinity of M87’s black hole (which I’ll call `M87bh’ for short) has not been challenged, and I’m not expecting it to be. But what and where exactly is the material that is emitting the radio waves and thus creating the glow in the image? And what exactly determines the size of the dark region at the center of the image? These have been problematic issues from the beginning, but discussion is starting to heat up. And it’s important: it has implications for the measurement of the black hole’s mass (which EHT claims is that of 6.5 billion Suns, with an uncertainty of about 15%), and for any attempt to estimate its rotation rate.
Over the last few weeks I’ve spent some time studying the mathematics of spinning black holes, talking to my Harvard colleagues who are world’s experts on the relevant math and physics, and learning from colleagues who produced the `photo’ and interpreted it. So I think I can now clearly explain what most journalists and scientist-writers (including me) got wrong at the time of the photo’s publication, and clarify what the photo does and doesn’t tell us.
One note before I begin: this post is long. But it starts with a summary of the situation that you can read quickly, and then comes the long part: a step-by-step non-technical explanation of an important aspect of the black hole ‘photo’ that, to my knowledge, has not yet been given anywhere else.
[I am heavily indebted to Harvard postdocs Alex Lupsasca and Shahar Hadar for assisting me as I studied the formulas and concepts relevant for fast-spinning black holes. Much of what I learned comes from early 1970s papers, especially those by my former colleague Professor Jim Bardeen (see this one written with Press and Teukolsky), and from papers written in the last couple of years, especially this one by my present and former Harvard colleagues.]
What Does the EHT Image Show?
Scientists understand the black hole itself — the geometric dimple in space and time — pretty well. If one knows the mass and the rotation rate of the black hole, and assumes Einstein’s equations for gravity are mostly correct (for which we have considerable evidence, for example from LIGO measurements and elsewhere), then the equations tell us what the black hole does to space and time and how its gravity works.
But for the `photo’, that’s not enough information. We don’t get to observe the black hole itself (it’s black, after all!) What the `photo’ shows is a blurry ring of radio waves, emitted from hot material (a plasma of mostly electrons and protons) somewhere around the black hole — material whose location, velocity, and temperature we do not know. That material and its emission of radio waves are influenced by powerful gravitational forces (whose details depend on the rotation rate of the M87bh, which we don’t know yet) and powerful magnetic fields (whose details we hardly know at all.) The black hole’s gravity then causes the paths on which the radio waves travel to bend, even more than a glass lens will bend the path of visible light, so that where things appear in the ‘photo’ is not where they are actually located.
The only insights we have into this extreme environment come from computer simulations and a few other `photos’ at lower magnification. The simulations are based on well-understood equations, but the equations have to be solved approximately, using methods that may or may not be justified. And the simulations don’t tell you where the matter is; they tell you where the material will go, but only after you make a guess as to where it is located at some initial point in time. (In the same sense: computers can predict the national weather tomorrow only when you tell them what the national weather was yesterday.) No one knows for sure how accurate or misleading these simulations might be; they’ve been tested against some indirect measurements, but no one can say for sure what flaws they might have.
However, there is one thing we can certainly say, and it has just been said publicly in a paper by Samuel Gralla, Daniel Holz and Robert Wald.
Two months ago, when the EHT `photo’ appeared, it was widely reported in the popular press and on blogs that the photo shows the image of a photon sphere at the edge of the shadow of the M87bh. (Instead of `shadow’, I suggested the term ‘quasi-silhouette‘, which I viewed as somewhat less misleading to a non-expert.)
Unfortunately, it seems these statements are not true; and this was well-known to (but poorly communicated by, in my opinion) the EHT folks. This lack of clarity might perhaps annoy some scientists and science-loving non-experts; but does this issue also matter scientifically? Gralla et al., in their new preprint, suggest that it does (though they were careful to not yet make a precise claim.)
The Photon Sphere Doesn’t Exist…
Indeed, if you happened to be reading my posts carefully when the `photo’ first appeared, you probably noticed that I was quite vague about the photon-sphere — I never defined precisely what it was. You would have been right to read this as a warning sign, for indeed I wasn’t getting clear explanations of it from anyone. Studying the equations and conversing with expert colleagues, I soon learned why: for a rotating black hole, the photon sphere doesn’t really exist.
But let’s first define what the photon sphere is for a non-rotating black hole! Like the Earth’s equator, the photon sphere is a location, not an object. This location is the surface of an imaginary ball, lying well outside the black hole’s horizon. On the photon sphere, photons (the particles that make up light, radio waves, and all other electromagnetic waves) travel on special circular or spherical orbits around the black hole.
By contrast, a rotating black hole has a larger, broader `photon-zone’ where photons can have special orbits. But you won’t ever see the whole photon zone in any image of a rotating black hole. Instead, a piece of the photon zone will appear as a `photon ring‘, a bright and very thin loop of radio waves. However, the photon ring is not the edge of anything spherical, is generally not perfectly circular, and generally is not even perfectly centered on the black hole.
… and the Photon Ring Isn’t What We See…
It seems likely that the M87bh is rotating quite rapidly, so it has a photon-zone rather than a photon-sphere, and images of it will have a photon ring. Ok, fine; but then, can we interpret EHT’s `photo’ simply as showing the photon ring, blurred by the imperfections in the `telescope’? Although some of the EHT folks have seemed to suggest the answer is “yes”, Gralla et al. suggest the answer is likely “no” (and many of their colleagues have been pointing out the same thing in private.) The circlet of radio waves that appears in the EHT `photo’ is probably not simply a blurred image of M87bh’s photon ring; it probably shows a combination of the photon ring with something brighter (as explained below). That’s where the controversy starts.
…so the Dark Patch May Not Be the Full Shadow…
The term `shadow’ is confusing (which is why I prefer `quasi-silhouette’ in describing it in public contexts, though that’s my own personal term) but no matter what you call it, in its ideal form it is supposed to be an absolutely dark area whose edge is the photon ring. But in reality the perfectly dark area need not appear so dark after all; it may be partly filled in by various effects. Furthermore, since the `photo’ may not show us the photon ring, it’s far from clear that the dark patch in the center is the full shadow anyway. The EHT folks are well aware of this, but at the time the photo came out, many science writers and scientist-writers (including me) were not.
…so EHT’s Measurement of the M87bh’s Mass is Being Questioned
It was wonderful that EHT could make a picture that could travel round the internet at the speed of light, and generate justifiable excitement and awe that human beings could indirectly observe such an amazing thing as a black hole with a mass of several billion Sun-like stars. Qualitatively, they achieved something fantastic in showing that yes, the object at the center of M87 really is as compact and dark as such a black hole would be expected to be! But the EHT telescope’s main quantitative achievement was a measurement of the mass of the M87bh, with a claimed precision of about 15%.
Naively, one could imagine that the mass is measured by looking at the diameter of the dark spot in the black hole ‘photo’, under the assumption that it is the black hole’s shadow. So here’s the issue: Could interpreting the dark region incorrectly perhaps lead to a significant mistake in the mass measurement, and/or an underestimate of how uncertain the mass measurement actually is?
I don’t know. The EHT folks are certainly aware of these issues; their simulations show them explicitly. The mass of the M87bh isn’t literally measured by putting a ruler on the ‘photo’ and measuring the size of the dark spot! The actual methods are much more sophisticated than that, and I don’t understand them well enough yet to explain, evaluate or criticize them. All I can say with confidence right now is that these are important questions that experts currently are debating, and consensus on the answer may not be achieved for quite a while.
———————————————————————-
The Appearance of a Black Hole With Nearby Matter
Ok, now I’m going to explain the most relevant points, step-by-step. Grab a cup of coffee or tea, find a comfy chair, and bear with me.
Because fast-rotating black holes are more complicated, I’m going to start illuminating the controversy by looking at a non-rotating black hole’s properties, which is also what Gralla et al. mainly do in their paper. It turns out the qualitative conclusion drawn from the non-rotating case largely applies in the rotating case too, at least in the case of the M87bh as seen from our perspective; that’s important because the M87bh may well be rotating at a very good clip.
A little terminology first: for a rotating black hole there’s a natural definition of the poles and the equator, just as there is for the Earth: there’s an axis of rotation, and the poles are where that axis intersects with the black hole horizon. The equator is the circle that lies halfway between the poles. For a non-rotating black hole, there’s no such axis and no such automatic definition, but it will be useful to define the north pole of the black hole to be the point on the horizon closest to us.
A Single Source of Electromagnetic Waves
Let’s imagine placing a bright light bulb on the same plane as the equator, outside the black hole horizon but rather close to it. (The bulb could emit radio waves or visible light or any other form of electromagnetic waves, at any frequency; for what I’m about to say, it doesn’t matter at all, so I’ll just call it `light’.) See Figure 1. Where will the light from the bulb go?
Some of it, heading inward, ends up in the black hole, while some of it heads outward toward distant observers. The gravity of the black hole will bend the path of the light. And here’s something remarkable: a small fraction of the light, aimed just so, can actually spiral around the black hole any number of times before heading out. As a result, you will see the bulb not once but multiple times!
There will be a direct image — light that comes directly to us — from near the bulb’s true location (displaced because gravity bends the light a bit, just as a glass lens will distort the appearance of what’s behind it.) That path of that light is the orange arrow in Figure 1. But then there will be an indirect image (the green arrow in Figure 1) from light that goes halfway around the black hole before heading in our direction; we will see that image of the bulb on the opposite side of the black hole. Let’s call that the `first indirect image.’ Then there will be a second indirect image from light that orbits the black hole once and comes out near the direct image, but further out; that’s the blue arrow in Figure 1. Then there will be a third indirect image from light that goes around one and a half times (not shown), and so on. In short, Figure 1 shows the paths of the direct, first indirect, and second indirect images of the bulb as they head toward our location at the top of the image.
In the case of a rotating black hole, something very similar happens when you’re looking at the black hole from its north (or south) pole; there’s a special circle then too. But that circle is not the edge of a photon-sphere! In general, photons can have special orbits in a wide region, which I called the “photon-zone” earlier, and only a small set of them are on this circle. You’ll see photons from other parts of the photon zone if you look at the black hole not from the poles but from some other angle.
[If you’d like to learn a bit more about the photon zone, and you have a little bit of knowledge of black holes already, you can profit from exploring this demo by Professor Leo Stein: https://duetosymmetry.com/tool/kerr-circular-photon-orbits/ ]
Back to the non-rotating case: What our camera will see, looking at what is emitted from the light bulb, is shown in Figure 2: an infinite number of increasingly squished `indirect’ images, half on one side of the black hole near the direct image, and the other half on the other side. What is not obvious, but true, is that only the first of the indirect images is large and bright; this is one of Gralla et al.‘s main points. We can, therefore, separate the images into the direct image, the first indirect image, and the remaining indirect images. The total amount of light coming from the direct image and the first indirect image can be large, but the total amount of light from the remaining indirect images is typically (according to Gralla et al.) less than 5% of the light from the first indirect image. And so, unless we have an extremely high-powered camera, we’ll never pick those other images up. Let’s therefore focus our attention on the direct image and the first indirect image.
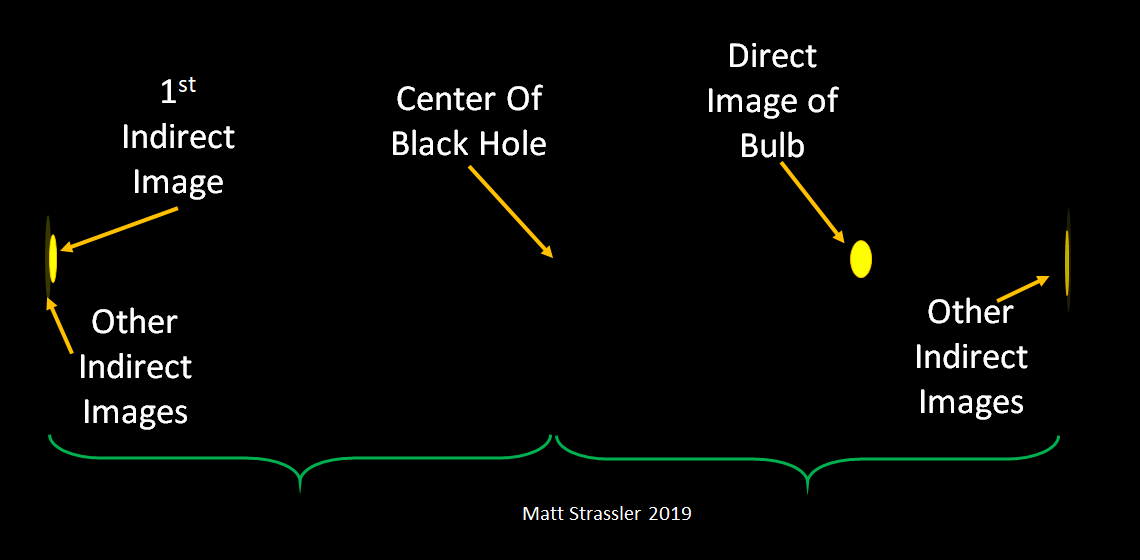
WARNING (since this seems to be a common confusion):
IN ALL MY FIGURES IN THIS POST, AS IN THE BLACK HOLE `PHOTO’ ITSELF, THE COLORS OF THE IMAGES ARE CHOSEN ARBITRARILY (as explained in my first blog post on this subject.) THE `PHOTO’ WAS TAKEN AT A SINGLE, NON-VISIBLE FREQUENCY OF ELECTROMAGNETIC WAVES: EVEN IF WE COULD SEE THAT TYPE OF RADIO WAVE WITH OUR EYES, IT WOULD BE A SINGLE COLOR, AND THE ONLY THING THAT WOULD VARY ACROSS THE IMAGE IS BRIGHTNESS. IN THIS SENSE, A BLACK AND WHITE IMAGE MIGHT BE CLEARER CONCEPTUALLY, BUT IT IS HARDER FOR THE EYE TO PROCESS.
A Circular Source of Electromagnetic Waves
Proceeding step by step toward a more realistic situation, let’s replace our ordinary bulb by a circular bulb (Figure 3), again set somewhat close to the horizon, sitting in the plane that contains the equator. What would we see now?
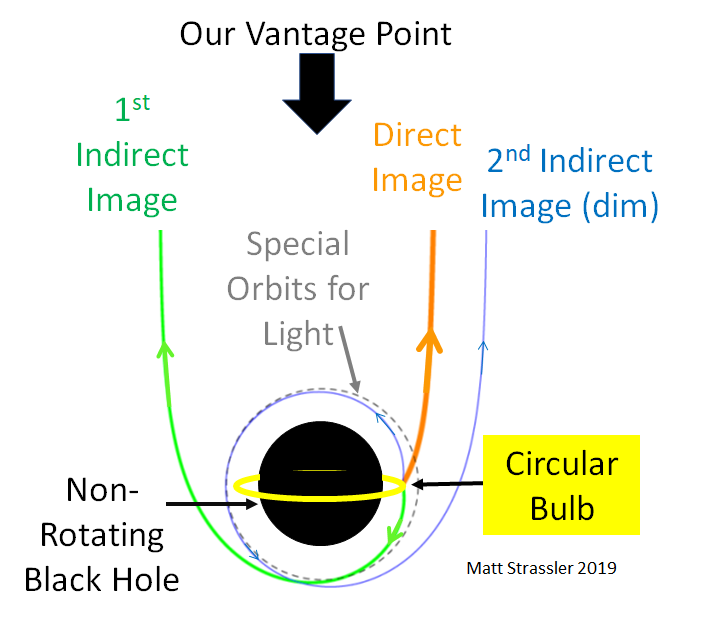
That’s shown in Figure 4: the direct image is a circle (looking somewhat larger than it really is); outside it sits the first indirect image of the ring; and then come all the other indirect images, looking quite dim and all piling up at one radius. We’re going to call all those piled-up images the “photon ring”.
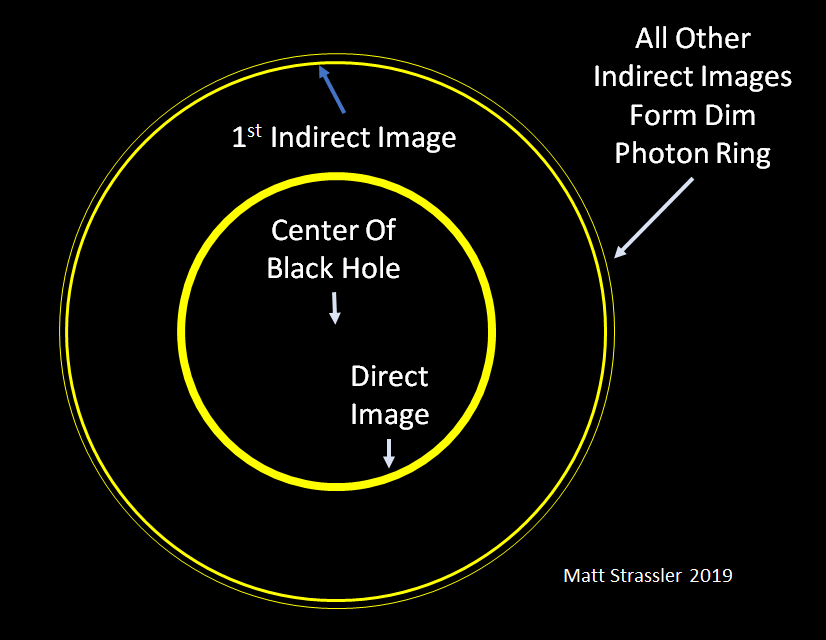
Importantly, if we consider circular bulbs of different diameter [yellow, red and blue in Figure 5], then although the direct images reflect the differences in the bulbs’ diameters (somewhat enlarged by lensing), the first indirect images all are about the same diameter, just a tad larger or smaller than the photon ring. The remaining indirect images all sit together at the radius of the photon ring.
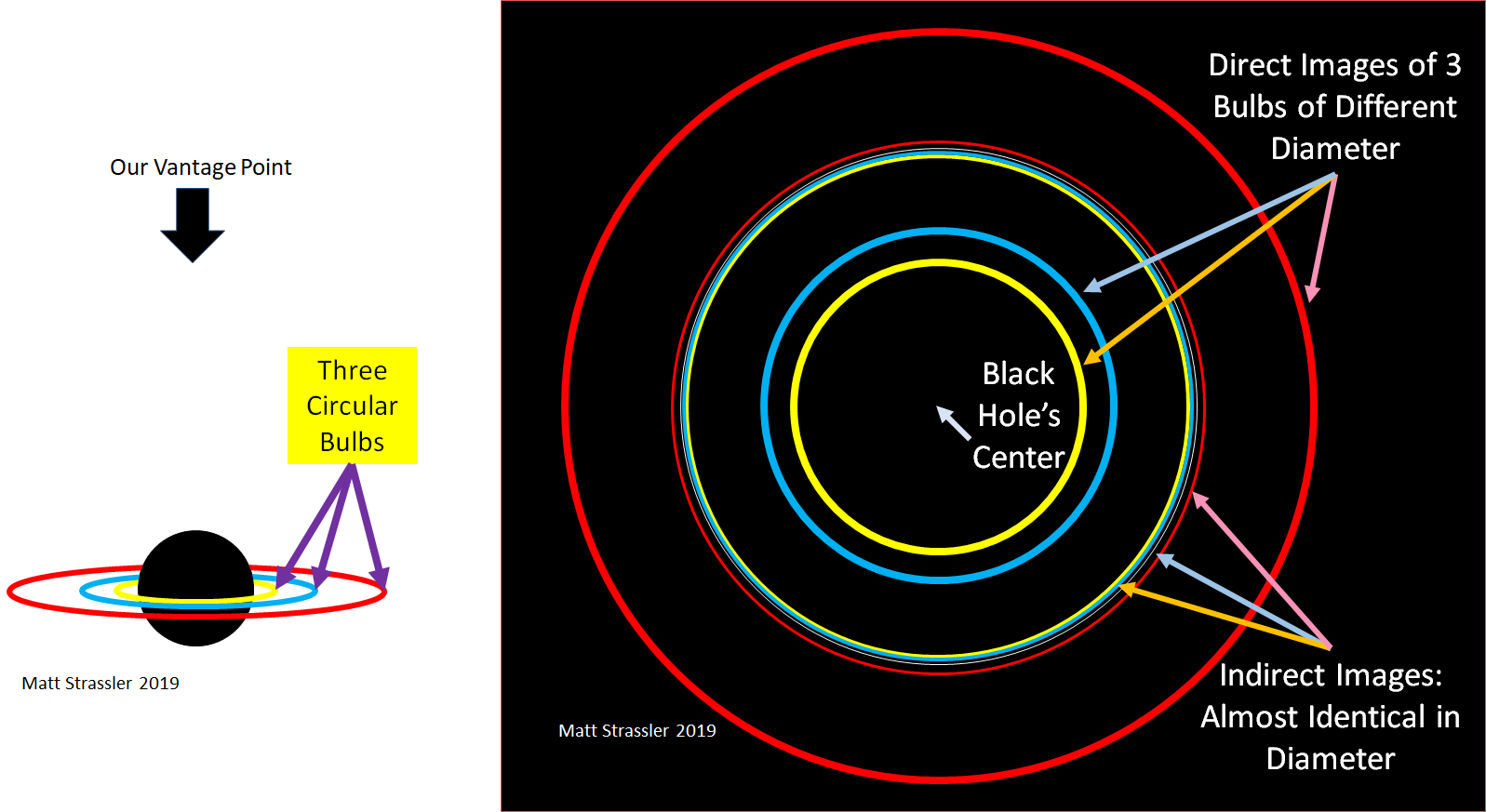
These statements are also essentially true for a rotating black hole seen from the north or south pole; a circular bulb generates a series of circular images, and the indirect images all pile more or less on top of each other, forming a photon ring. When viewed off the poles, the rotating black hole becomes a more complicated story, but as long as the viewing angle is small enough, the changes are relatively minor and the picture is qualitatively somewhat similar.
A Disk as a Source of Electromagnetic Waves
And what if you replaced the circular bulb with a disk-shaped bulb, a sort of glowing pancake with a circular hole at its center, as in Figure 7? That’s relevant because black holes are thought to have `accretion disks’ made of material orbiting the black hole, and eventually spiraling in. The accretion disk may well be the dominant source emitting radio waves at the M87bh. (I’m showing a very thin uniform disk for illustration, but a real accretion disk is not uniform, changes rapidly as clumps of material move within it and then spiral into the black hole, and may be quite thick — as thick as the black hole is wide, or even thicker.)
Well, we can think of the disk as many concentric circles of light placed together. The direct images of the disk (shown in Figure 6 left, on one side of the disk, as an orange wash) would form a disk in your camera, the dim red region in Figure 6 right; the hole at its center would appear larger than it really is due to the bending caused by the black hole’s gravity, but the shape would be similar. However, the indirect images would all pile up in almost the same place from your perspective, forming a bright and quite thin ring, the bright yellow circle in Figure 6 right. (The path of the disk’s first indirect image is shown in Figure 6 left, going halfway about the black hole as a green wash; notice how it narrows as it travels, which is why it appears as a narrow ring in the image at right.) This circle — the full set of indirect images of the whole disk — is the edge of the photon-sphere for a non-rotating black hole, and the circular photon ring for a rotating black hole viewed from its north or south pole.
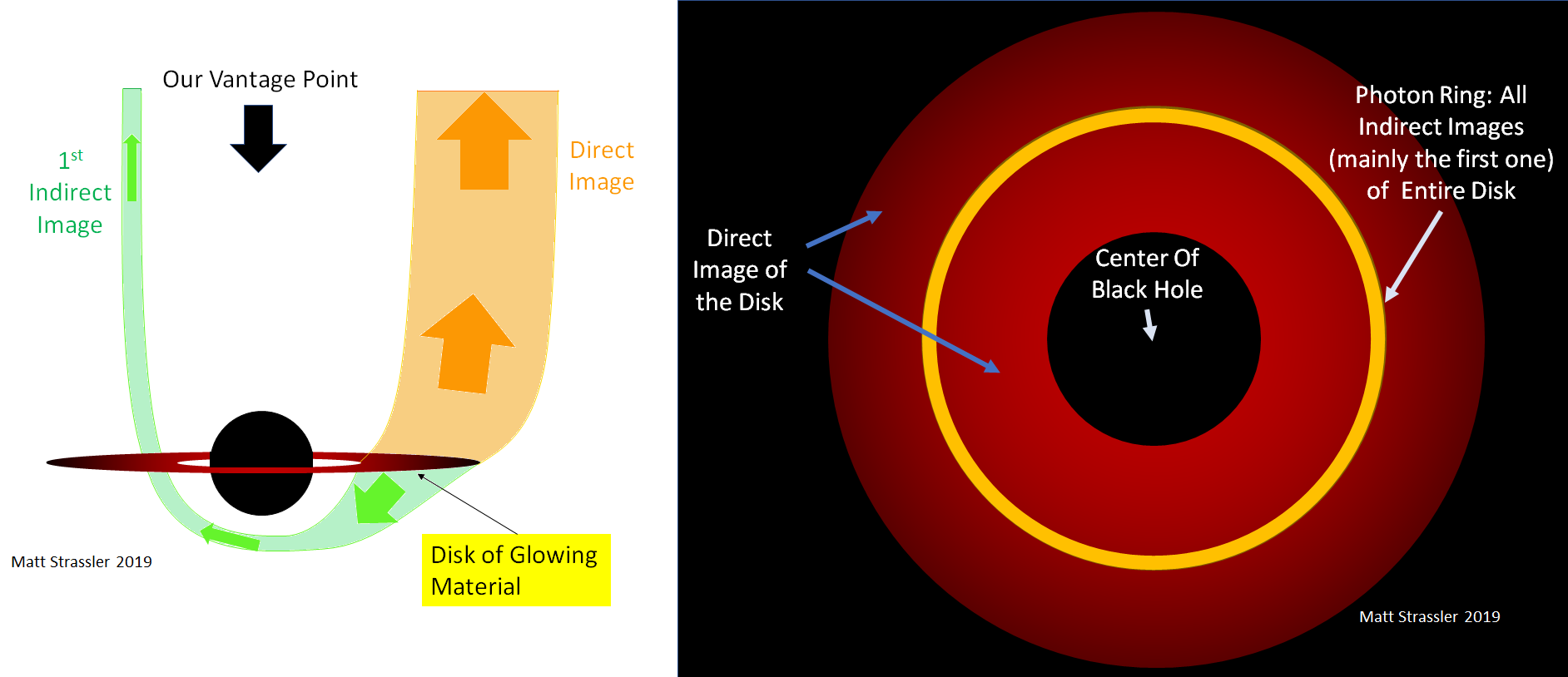
[Gralla et al. call the first indirect image the `lensed ring’ and the remaining indirect images, currently unobservable at EHT, the `photon ring’, while EHT refers to all the indirect images as the `photon ring’. Just letting you know in case you hear `lensed ring’ referred to in future.]
So the conclusion is that if we had a perfect camera, the direct image of a disk makes a disk, but the indirect images (mainly just the first one, as Gralla et al. emphasize) make a bright, thin ring that may be superposed upon the direct image of the disk, depending on the disk’s shape.
And this conclusion, with some important adjustments, applies also for a spinning black hole viewed from above its north or south pole — i.e., along its axis of rotation — or from near that axis; I’ll mention the adjustments in a moment.
But EHT is not a perfect camera. To make the black hole image, technology had to be pushed to its absolute limits. Someday we’ll see both the disk and the ring, but right now, they’re all blurred together. So which one is more important?
From a Blurry Image to Blurry Knowledge
What does a blurry camera do to this simple image? You might think that the disk is so dim and the ring so bright that the camera will mainly show you a blurry image of the bright photon ring. But that’s wrong. The ring isn’t bright enough. A simple calculation reveals that the photo will show mainly the disk, not the photon ring! This is shown in Figure 9, which you can compare with the Black Hole `photo’ (Figure 10). (Figure 9 is symmetric around the ring, but the photo is not, for multiple reasons — Doppler-like effect from rotation, viewpoint off the rotation axis, etc. — which I’ll have to defer til another post.)
More precisely, the ring and disk blur together, but the brightness of the image is dominated by the disk, not the ring.
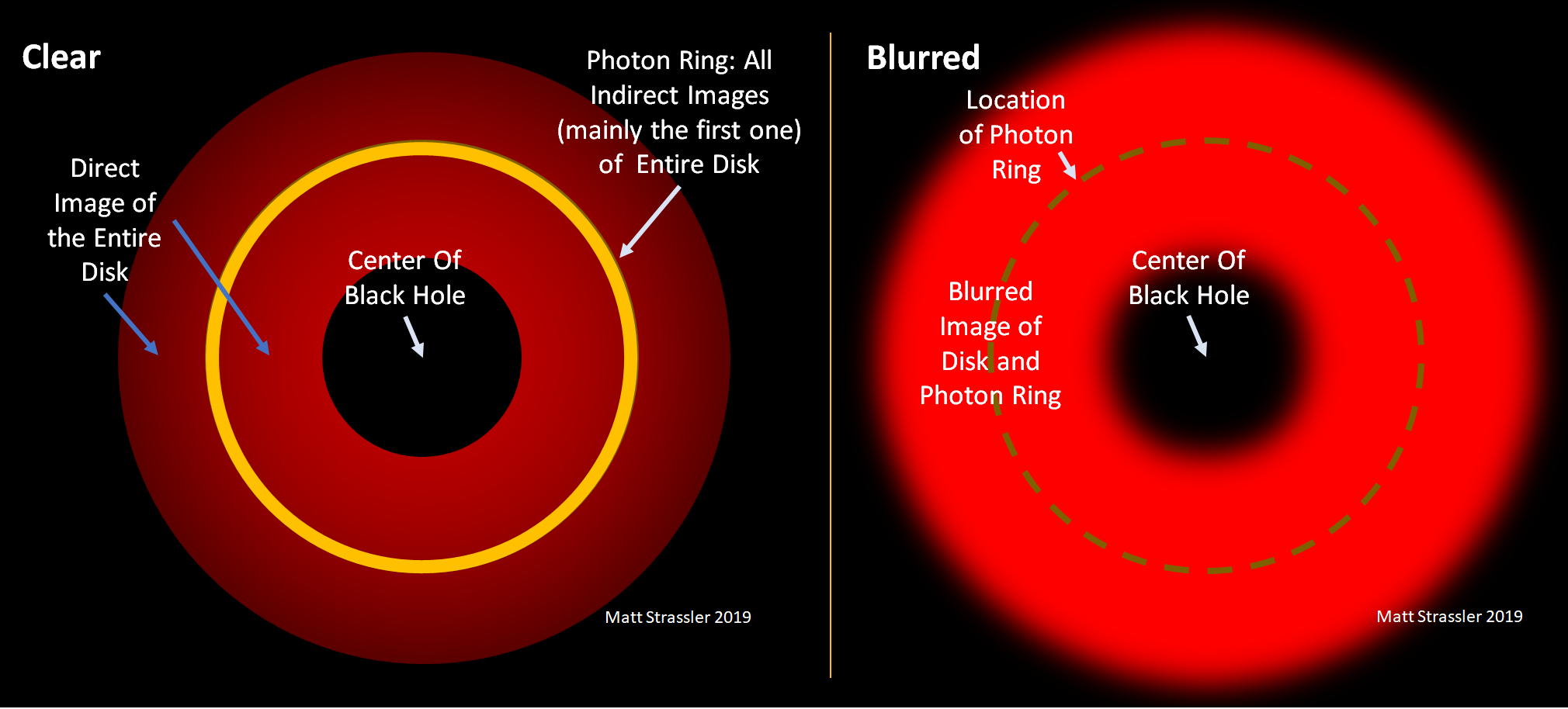
Let’s say that again: the black hole `photo’ may mainly show the M87bh’s accretion disk, with the photon ring contributing only some of the light, and therefore the photon ring does not completely and unambiguously determine the radius of the observed dark patch in the `photo.’ In general, the patch could be considerably smaller than what is usually termed the `shadow’ of the black hole.
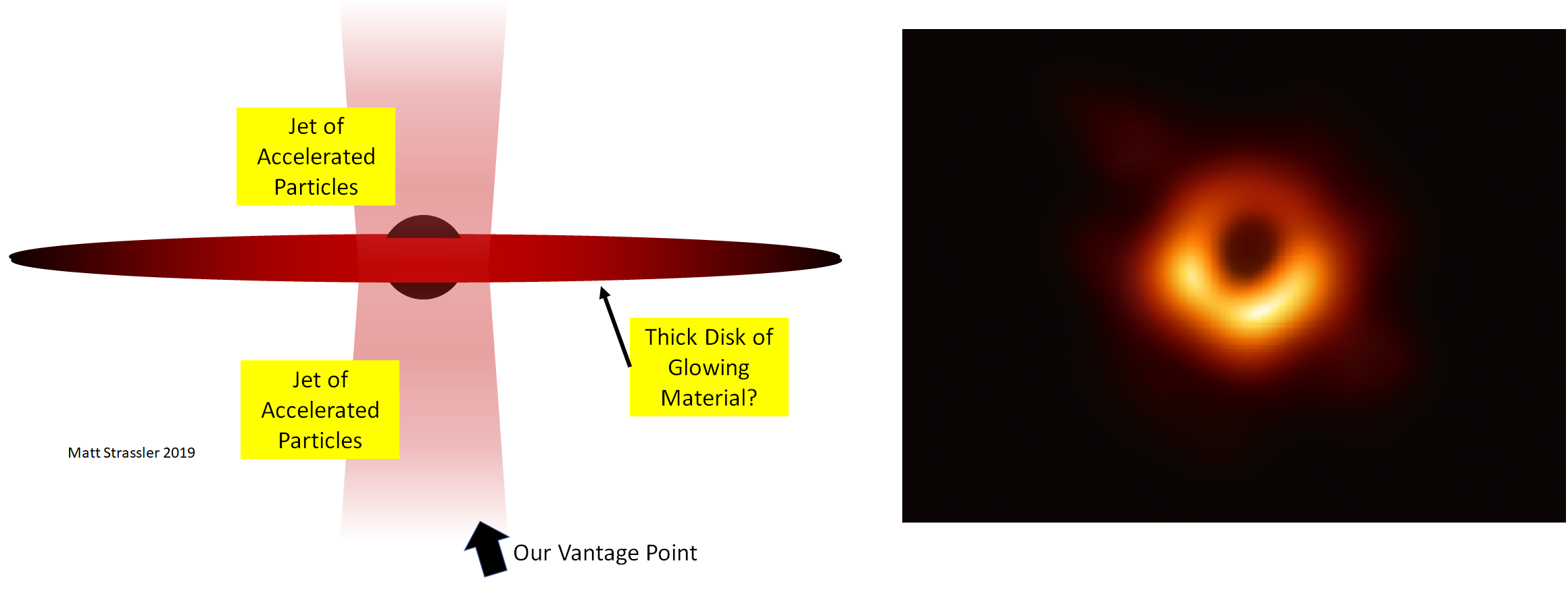
This is important. The photon ring’s diameter, and thus the width of the `shadow’ too, barely depend on the rotation rate of the black hole; they depend almost exclusively on the black hole’s mass. So if the ring in the photo were simply the photon ring of the M87bh, you’d have a very simple way to measure the black hole’s mass without knowing its rotation rate: you’d look at how large the dark patch is, or equivalently, the diameter of the blurry ring, and that would give you the answer to within 10%. But it’s nowhere near so simple if the blurry ring shows the accretion disk, because the accretion disk’s properties and appearance can vary much more than the photon ring; they can depend strongly on the black hole’s rotation rate, and also on magnetic fields and other details of the black hole’s vicinity.
The Important Role of Rotation
If we conclude that EHT is seeing a mix of the accretion disk with the photon ring, with the former dominating the brightness, then this makes EHT’s measurement of the M87bh’s mass more confusing and even potentially suspect. Hence: controversy. Is it possible that EHT underestimated their uncertainties, and that their measurement of the black hole mass has more ambiguities, and is not as precise, as they currently claim?
Here’s where the rotation rate is important. Despite what I showed (for pedagogical simplicity) in Figure 7, for a non-rotating black hole the accretion disk’s central gap is actually expected to lie outside the photon ring; this is shown at the top of Figure 9. But the faster the black hole rotates, the smaller this central gap is expected to be, to the point that for a fast-rotating black hole the gap will lie inside the photon ring, as shown at the bottom of Figure 9. (This tendency is not obvious; it requires understanding details of the black hole geometry.) And if that is true, the dark patch in the EHT image may not be the black hole’s full shadow (i.e. quasi-silhouette), which is the region inside the photon ring. It may be just the inner portion of it, with the outer portion obscured by emission from the accretion disk.
The effect of blurring in the two cases of slow (or zero) and fast rotation are illustrated in Figure 9, where the photon ring’s size is taken to be the same in each case but the disk’s inner edge is close in or far out. (The black holes, not illustrated since they aren’t visible anyway, differ in mass by about 10% in order to have the photon ring the same size.) This shows why the size of the dark patch can be quite different, depending on the disk’s shape, even when the photon ring’s size is the same.
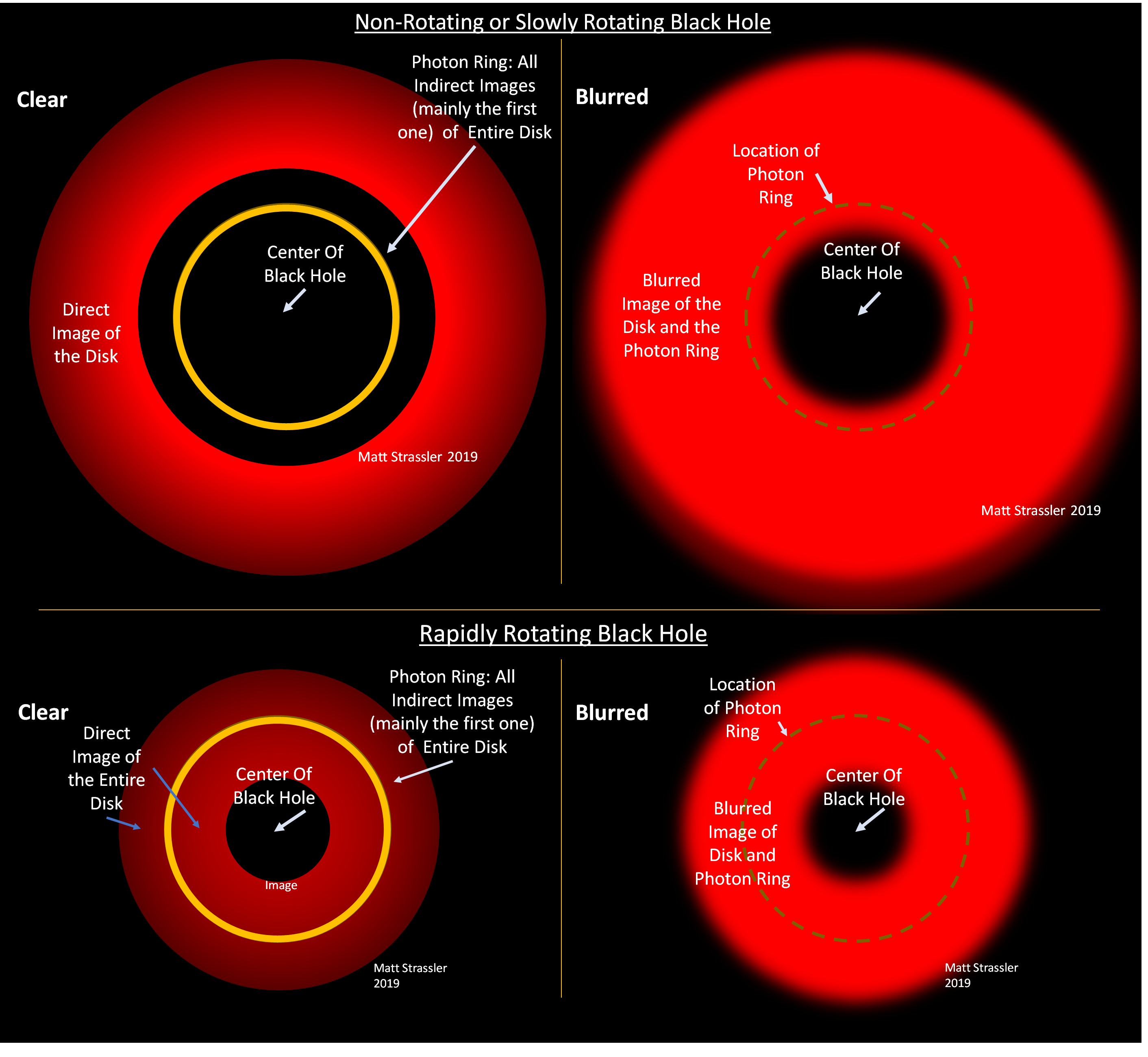
Gralla et al. subtly raise these questions but are careful not to overstate their case, perhaps because they have not yet completed their study of rotating black holes. But the question is now in the air.
I’m interested to hear what the EHT folks have to say about it, as I’m sure they have detailed arguments in favor of their procedures. In particular, EHT’s simulations show all of the effects mentioned above; there’s none of this of which they are unaware. (In fact, the reason I know my illustrations above are reasonable is partly because you can see similar pictures in the EHT papers.) As long as the EHT folks correctly accounted for all the issues, then they should have been able to properly measure the mass and estimate their uncertainties correctly. In fact, they don’t really use the photo itself; they use more subtle techniques applied to their telescope data directly. Thus it’s not enough to argue the photo itself is ambiguous; one has to argue that EHT’s more subtle analysis methods are flawed. No one has argued that yet, as far as I am aware.
But the one thing that’s clear right now is that science writers almost uniformly got it wrong [because the experts didn’t explain these points well] when they tried to describe the image two months ago. The `photo’ probably does not show “a photon ring surrounding a shadow.” That would be nice and simple and impressive-sounding, since it refers to fundamental properties of the black hole’s warping effects on space. But it’s far too glib, as Figures 7 and 9 show. We’re probably seeing an accretion disk supplemented by a photon ring, all blurred together, and the dark region may well be smaller than the black hole’s shadow.
(Rather than, or in addition to, the accretion disk, it is also possible that the dominant emission in the photo comes from the inner portion of one of the jets that emerges from the vicinity of the black hole; see Figure 8 above. This is another detail that makes the situation more difficult to interpret, but doesn’t change the main point I’m making.)
Someday in the not distant future, improved imaging should allow EHT to separately image the photon ring and the disk, so both can be observed easily, as in the left side of Figure 9. Then all these questions will be answered definitively.
Why the Gargantua Black Hole from Interstellar is Completely Different
Just as a quick aside, what would you see if an accretion disk were edge-on rather than face-on? Then, in a perfect camera, you’d see something like the famous picture of Gargantua, the black hole from the movie Interstellar — a direct image of the front edge of the disk, and a strongly lensed indirect image of the back side of the disk, appearing both above and below the black hole, as illustrated in Figure 11. And that leads to the Gargantua image from the movie, also shown in Figure 11. Notice the photon ring (which is, as I cautioned you earlier, off-center!) [Note added: this figure has been modified; in the original version I referred to the top and bottom views of the disk’s far side as the “1st indirect image”, but as pointed out by Professor Jean-Pierre Luminet, that’s not correct terminology here.]
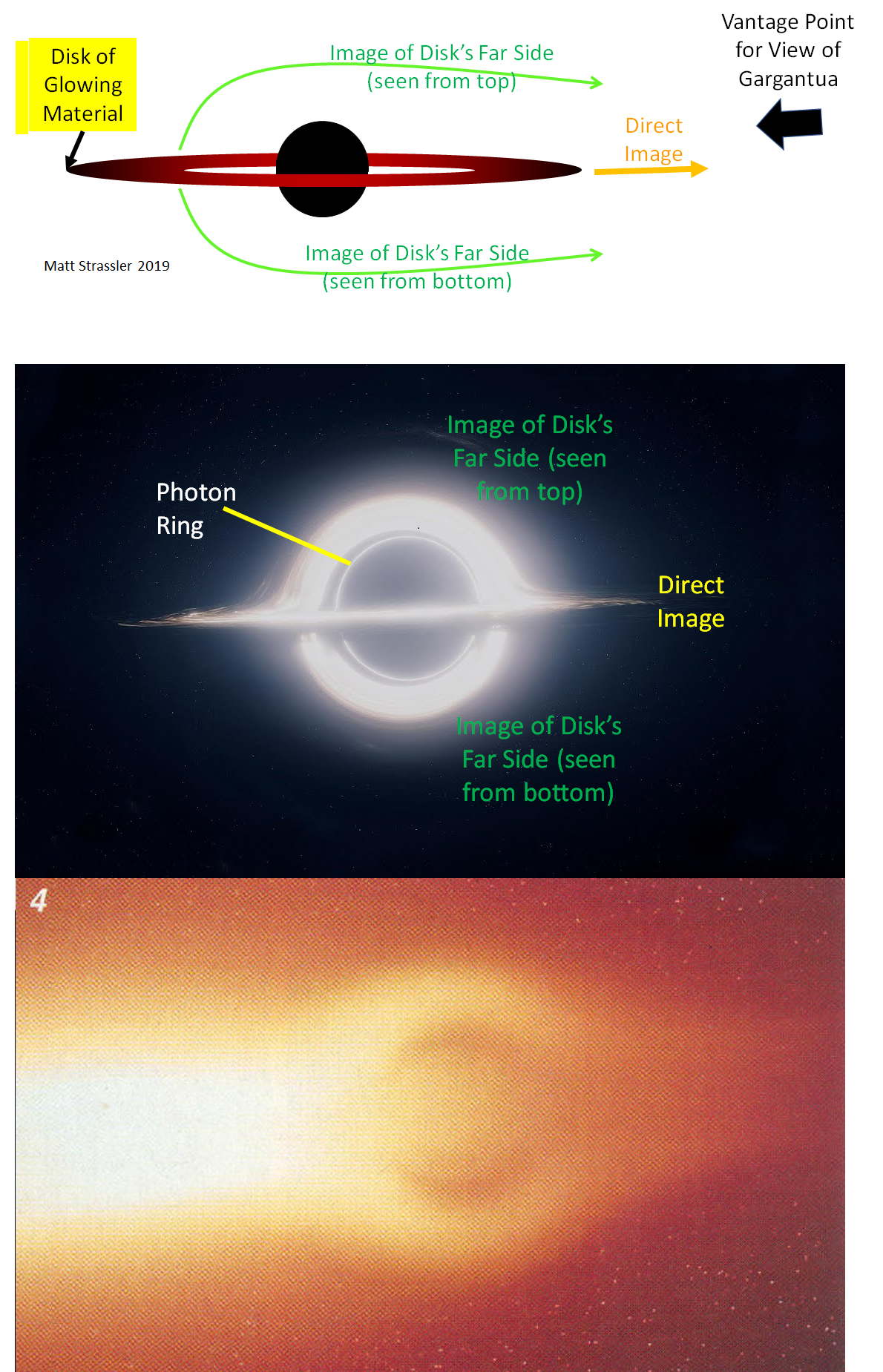
However, the movie image leaves out an important Doppler-like effect (which I’ll explain someday when I understand it 100%). This makes the part of the disk that is rotating toward us bright, and the part rotating away from us dim… and so a real image from this vantage point would be very asymmetric — bright on the left, dim on the right — unlike the movie image. At the suggestion of Professsor Jean-Pierre Luminet I have added, at the bottom of Figure 10, a very early simulation by Jean-Alain Marck that shows this effect.
I mention this because a number of expert science journalists incorrectly explained the M87 image by referring to Gargantua — but that image has essentially nothing to do with the recent black hole `photo’. M87’s accretion disk is certainly not edge-on. The movie’s Gargantua image is taken from the equator, not from near the pole.
Final Remarks: Where a Rotating Black Hole Differs from a Non-Rotating One
Before I quit for the week, I’ll just summarize a few big differences for fast-rotating black holes compared to non-rotating ones.
1) As I’ve just emphasized, what a rotating black hole looks like to a distant observer depends not only on where the matter around the black hole is located but also on how the black hole’s rotation axis is oriented relative to the observer. A pole observer, an equatorial observer, and a near-pole observer see quite different things. (As noted in Figure 8, we are apparently near-south-pole observers for M87’s black hole.)
Let’s assume that the accretion disk lies in the same plane as the black hole’s equator — there are some reasons to expect this. Even then, the story is complex.
2) As I mentioned above, instead of a photon-sphere, there is a ‘photon-zone’ — a region where specially aimed photons can travel round the black hole multiple times. For high-enough spin (greater than about 80% of maximum as I recall), an accretion disk’s inner edge can lie within the photon zone, or even closer to the black hole than the photon zone; and this can cause a filling-in of the ‘shadow’.
3) Depending on the viewing angle, the indirect images of the disk that form the photon ring may not be a circle, and may not be concentric with the direct image of the disk. Only when viewed from along the rotation axis (i.e., above the north or south pole) will the direct and indirect images of the disk all be circular and concentric. We’re not viewing the M87bh on its axis, and that further complicates interpretation of the blurry image.
4) When the viewing angle is not along the rotation axis the image will be asymmetric, brighter on one side than the other. (This is true of EHT’s `photo’.) However, I know of at least four potential causes of this asymmetry, any or all of which might play a role, and the degree of asymmetry depends on properties of the accretion disk and the rotation rate of the black hole, both of which are currently unknown. Claims about the asymmetry made by the EHT folks seem, at least to me, to be based on certain assumptions that I, at least, cannot currently check.
Each of these complexities is a challenge to explain, so I’ll give both you and I a substantial break while I figure out how best to convey what is known (at least to me) about these issues.
50 Responses
A realistic picture of “Gargantua” (with the effect of Doppler shift of light emitted by the rotating disk) can be found in the paper published by the “Interstellar” visualisations team: https://doi.org/10.1088/0264-9381/32/6/065001
Thanks for the detailed and exceptionally clear post on this sometimes confusing discovery / scientific debate. I especially liked the progression of more and more sophisticated light sources culminating in why we would expect to see the photon ring if we had high-enough resolution.
I’m still left with a question about interpreting the image you may be able to help with: how aligned would the accretion disk be with the rotation plane of the black hole? I would imagine that if they were not perfectly aligned you’d have both increased assymetry in the image, and have the middle of the image much farther away from the center of the system.
The connection between the plane of the accretion disk and the rotation plane of the black hole is not obvious at all. I’m not able to give a informed answer yet about what is and isn’t known, but I can give you my present understanding. Simulations do not show that the planes must be the same. If the black hole is huge and ancient and the disk new, they could be uncorrelated; if, on the other hand, the disk is old and has been fueling the black hole for a long time, then its rotation may now be imprinted on the black hole. Also, there are some effects that can tend to orient the disk along the black hole’s equator, but these effects do not necessarily act rapidly. One of these was in the news recently, though I can’t attest to the accuracy or clarity of the article: https://www.universetoday.com/142453/black-hole-simulation-solves-a-mystery-about-their-accretion-disks/ .
This video explains very clearly how the photons are compressed near a BH and takes inspiration from the indications of the professor Luminet. https://www.youtube.com/watch?v=zUyH3XhpLTo
Thanks for the update! It took me several hours the first couple of days to understand that the photon sphere did not exist, the topology of top view vs other viewing angles et cetera. (Not from math, just from authoritative voices – I haven’t studied GR.)
If I remember the papers correctly, it is the intensity asymmetry that you mention at the very end that allows the EHT estimate – IIRC they claim the rotation is the likeliest cause (for such reasons that the hole likely rotates, et cetera). If the controversy is simply that it is early days et cetera, it isn’t much to argue about if they want to use the Sag A* or the future larger telescope as sanity check (which I think they noted), they have the best estimate for the time being. And not being involved in the area, it is easy to wait while the professional strife for glory settles.
Professor Luminet cites his article to clarify any inaccuracies that I honestly do not have the skills to evaluate, I would like to understand which ones are the most exact. https://arxiv.org/ftp/arxiv/papers/1902/1902.11196.pdf
So far, aside from this one error [which I have already corrected above, and was more a poor choice of terminology than a physics issue], Professor Luminet only seems to be complaining that I didn’t get the history correct. But I made no attempt to review the history in this post.
Professor I tagged you on my facebook page where there are also objections from professor Luminet.https://www.facebook.com/fabrizio.kasto/posts/2637818456248247?comment_id=2640285676001525¬if_id=1560640171257612¬if_t=feedback_reaction_generic
That’s fine, but I still see no errors other than the primary/secondary image. Professor Luminet refers to errors, plural, but so far I only see one, singular. If you are aware of other errors, please let me know before I make the changes to this post. Obviously I’m happy to give Professor Luminet some credit, but his attitude toward a non-expert is not kind.
Note also that the fact that simulations had been done earlier (better or worse) is irrelevant to this post, which is not trying to set history straight. This is not a document about the history of the field, nor does it pretend to be, and no one is cited except for the most recent activity and papers that I personally learned from.
/The singularity inside an non-rotating black hole is really not a point in space; it is a moment in time!/
A rotating black hole cannot form singularity, but only unlike stars, planets, drag space with its rotation?
The gravitational time dilation around black hole carry information about the black hole mass, under equivalence principle of matter waves and electromagnetic waves. But singularity block this “rest mass” information or “no equivalence principle”?
Moment in time = No momentum in space (no rotation) = No “rest mass (unlike the time dilation, a phenomenology under equivalence principle)”?
Thanks for writing this.
I believe professor should make corrections in his article as Professor Luminet suggests : for instance his description of the image from Interstellar is wrong. The direct (primary) image of the top of the disk is only the central and upper part, the secundary image of the bottom is the lower part. Now a much better simulation including all the physical properties of the disk (intrinsic luminosity, Doppler shift, diffusion effects) had been calculated in 1991
Sorry, I do not understand precisely how you mean this, but am happy to make a correction if I have an error. Could you point me to Professor Luminet’s comment? Also, do you disagree with the arrows in the upper diagram in Figure 10, or simply with the terminology “indirect image”?
Thanks for your excellent article.
Figure 7 illustrates a non rotating black hole, but figure 9 shows it as the lower image and is labeled as a rotating black hole. Is figure 9 labelled correctly?
Please tell me if I’m wrong.
Oh! Excellent point, and a serious lapse on my part in my explanation. Figure 9 is labelled correctly. Up to Figure 7 I am still taking illustrative and unrealistic examples, choosing the disk’s shape for convenience; but in Figure 9 I’m trying to explain how realistic examples work. (Disks with narrow gaps and non-rotating black holes are simpler to think about, but in the realistic case they don’t happen at the same time. It’s a pedagogical problem.) I did not have a statement that Figure 7 is unrealistic when I switched to Figure 9, and I definitely should have one… in fact an earlier version had one and as I rewrote the article I took it out. Mea culpa.
Reblogged this on In the Dark and commented:
This is a long post but well worth reading if you want to know what we really know about the black hole in M87..
Thanks!
Hi professor.
In the example of the lamp, there is there stretching of matter in the ring, (Einstein’s relativity)? Then, can we admit that the described image of the ring is real or not? In that case, is the EHT image real or not? In other words, is this thread format of BH, in it ring’s dimensions and proportions and its center, is there an reality or a gravitational lens effect on itself, a real image or stretched or disproportionate? I do not ask if is it blurred, but ask about dimensions and proportions, an fact that would determine their mass and rotation speed, data very important in calculations, because in the EHT photo you see many disproportions and swellings in the ring, where you say that is a nonuniform ring.
But then I ask if there would be in that lack of uniformity, different gravity in each part of the ring? there is the one explanation for this unevenness, or not? Lets not forget that this nonuniform can alter spins and moment of BH itself and its magnetic field. Finally, why would the BH extreme gravity allow you to receive direct image as you pointed? Is That no contradict the existence of indirect images and would violate the relativity and gravitational lens effect? Especially being on the edge of a BH? That I ask, would not you have to review your beautiful pictures of the text about diretc images? thanks
Furthermore, the mass of the material in the disk is tiny compared to the mass of the black hole, so that the non-uniformities in the ring will have little more effect on the black hole than a space station has on the Earth. And as you see in my figures the black hole’s gravity is *not* so extreme as you think. It can only block the images of objects inside its horizon. Objects outside the horizon can be seen directly, though their location is distorted by the bending of light by the black hole’s gravity.
You said “location is distorted” like sea waves? With external objects/mass reacting on it? thanks
Remember what you’re seeing in the actual black hole photo is a DISK of hot material, not an imaginary circular lamp. The material is gas-like and does not stretch anymore than air would stretch. And according to simulations, the disk tends to be highly non-uniform, rotates rapidly, and changes rapidly (on the time scale of a couple of days.)
Thanks master, last question: can we admiting that, if objects/mass spins around BH and BH spins too, then the gravitation field around, spins too? Then, inexist BH static?
And that would lead to all systems being spinning, planets, stars, galaxies, etc, only because their gravitational field is also spinning
Correcting: And would that lead to all systems being spinning, planets, stars, galaxies, etc, only because their gravitational field is also spinning? If That correct, lead to many things, many analyzes. Thats the question. thanks
No, objects like the Earth spin just as you would expect: because they are made of spinning material. The Earth and Sun are not nearly compact enough to cause space to rotate. A rotating black hole is what is left over when spinning material collapses in on itself, leaving behind only its gravity, including the rotation of space around it. [This is imprecise language; the correct way to say it involves getting into the equations, which is beyond what I can do here.]
Hi Matt, How’s it going? Is it true that the inside of a rotating black hole is a one dimensional line and not a singularity? Cheers Asani
Sent from my iPhone
>
Technically speaking, the singularity inside a rotating black hole is a ring. But this is more subtle than it sounds. In fact, the singularity inside an non-rotating black hole is really not a point in space; it is a moment in time! My understanding of black holes is not deep enough to figure out how explain these issues properly at the moment in a non-technical fashion; you may find more success with Sean Carroll, whose an expert on general relativity and a clear writer.
/”whose”/,
who’s
The word should be “who’s”.
Thanks for pointing out the typo.
Reading this made me feel the excitement of first seeing that ‘photo’ all over again! Thank you.
… I am curious if they were just lucky that ‘M87bh’ is oriented face-on. I wonder, were there previous other candidates where the ‘silhouette’ was obscured by the accretion disk? Or maybe clues from the surrounding environment the orientation? There’s so much I want to know now that black holes seem so ‘tangible!’
Reading this made me feel the excitement of first seeing that ‘photo’ all over again! Thank you.
… I am curious if they were just lucky that ‘M87bh’ is oriented face-on. I wonder, were there previous other candidates where the ‘silhouette’ was obscured by the accretion disk? Or maybe clues from the surrounding environment the orientation? There’s so much I want to know now that black holes seem so ‘tangible!’
The orientation is pure chance; there will be other black holes seen eventually with other orientations. This is the first time anyone’s looked for anything like this, because the methods available weren’t powerful enough. (And yes! isn’t it amazing to see the effects of these black holes so directly?)
This is great. One thing I’ve always wondered a bit about is what it really means to say a black hole is rotating, if the matter inside is collapsed into a singularity? How does it pick up the rotation–does it happen when the matter that originally collapses is rotating, and can the rotation speed or axis change as matter falls into the black hole?
The material that initially forms the black hole may be rotating — for example, essentially all stars rotate, and so a star that collapses to form a black hole will continue to rotate. Any other objects that fall in later can increase or decrease the spin rate (and change the axis of rotation). The practical effect of the rotation of the black hole is that it drags space around with it, making it impossible to stand still if you’re close enough to the black hole’s horizon (the point of no return.) The rotation of the space is very much measurable and meaningful. Let me see if I can find some good links about it.
Matt, Thanks for your clear explanation of what we see when we look at a black hole. There’s one other minuscule component that I haven’t seen mentioned. It occurred to me that since light can be bent at such large angles, there exists a path for the light from every star in the universe to bend around a black hole and end up striking the earth. Since black holes are so small only a small amount of light from each star is captured and deflected but it’s kinda cool to think that some of what we’re seeing is an image of everything.
Matt, I’m blown away by your illustrations. Must’ve taken quite a while to create them. Very nice explanation as well. Good job, and it’s good to see you posting more regularly now.
Congratulation about the article: finally someone approaching this subject in detail for the public, mainly about rises the light effect in the ring of the BH, facts rarely seen in scientific publications. And we want more of that, obscure points, like which an spins effects at BH and it ring, or if one makes the other spins. Thanks and we want more…
I enjoyed your article very much, I have some questions I’d like ask for clarification.
In Figure 7, the light forming the first indirect image seems to be traveling through the disc itself. Am I correctly interpreting that to mean that the disc is transparent to its own emitted light? In that case, I’m guessing that is what is meant by the terms “optically thin disk” I see in various papers or articles published by experts in this area?
In the section “The important role of rotation”, illustrated by Figure 9, it shows that the inner gap size changes depending on the black hole’s rotation rate. Is the gap size to be interpreted as the true size or apparent size? Meaning the size does actually physically change or is the size the same regardless of the rotation rate and only apparently changes due to light ray paths changing because of the rotation?
For my clarification, the reason for the gap between the edge of the accretion disk and the event horizon is due to inability for particles with mass to maintain stable orbits? So the inside edge of the disk represents the point of the last possible stable orbit, once a particle spirals past that point, it will inevitably fall into the black hole?
Good questions, deserving of comment within the post.
1) Optically thin does indeed mean that radio waves get through. I don’t think it’s automatic that these disks are optically thin (and it depends on the frequency of the radio waves.) Indeed the jets are not always optically thin and there was some worry, before the photo was taken, that they’d obscure the photon ring and the dark patch completely.
2) and 3) The gap size changes in reality; it’s not a geometric effect. And it is the same reason as the answer to your third question: there is a gap because when you get too close there are no longer any stable roughly-circular orbits, but the instability sets in closer for fast-rotating black holes than for slow-rotating ones. That requires a calculation, though not a hard one; you can find it mentioned in the Bardeen, Press and Teukolsky paper I cited. Your “inevitably” suggestion is too strong, however: a particle in that region is still outside the horizon, so it could, for instance, be steered by magnetic fields and flung out along one of the jets.
So the doppler effect ( in brightness ) is still seen even though the ‘photo’ is taken at a single frequency. Presumably dominated by light from the direct image.
Looking forward to your future post explaining it 🙂
This is precisely why I haven’t tried to explain it yet. The intrinsic brightness of the disk varies with frequency, and then the Doppler effect in this context affects the observed frequency certainly and the magnification as well (a geometric effect) so it’s not a simple matter to figure out how these interplay. I don’t yet even understand what the appropriate equations are, so I’m certainly not at the point where I could explain it to myself, much less to anyone else.
Many thanks Matt!