[Note added April 16: some minor improvements have been made to this article as my understanding has increased, specifically concerning the photon-sphere, which is the main region from which the radio waves are seen in the recently released image. See later blog posts for the image and its interpretation.]
[Note added June 14: significant misconceptions concerning the photon-sphere and shadow, as relevant to the black hole ‘photo’, dominated reporting in April, and I myself was also subject to them. I have explained the origin of and correction to these misconceptions, which affect the interpretation of the image, in my post “A Ring of Controversy”.]
About fifteen years ago, when I was a professor at the University of Washington, the particle physics theorists and the astronomer theorists occasionally would arrange to have lunch together, to facilitate an informal exchange of information about our adjacent fields. Among the many enjoyable discussions, one I was particularly excited about — as much as an amateur as a professional — was that in which I learned of the plan to make some sort of image of a black hole. I was told that this incredible feat would likely be achieved by 2020. The time, it seems, has arrived.
The goal of this post is to provide readers with what I hope will be a helpful guide through the foggy swamp that is likely to partly obscure this major scientific result. Over the last days I’ve been reading what both scientists and science journalists are writing in advance of the press conference Wednesday morning, and I’m finding many examples of jargon masquerading as English, terms poorly defined, and phrasing that seems likely to mislead. As I’m increasingly concerned that many non-experts will be unable to understand what is presented tomorrow, and what the pictures do and do not mean, I’m using this post to answer a few questions that many readers (and many of these writers) have perhaps not thought to ask.
A caution: I am not an expert on this subject. At the moment, I’m still learning about the more subtle issues. I’ll try to be clear about when I’m on the edge of my knowledge, and hopefully won’t make any blunders [but experts, please point them out if you see any!]
Which black holes are being imaged?
The initial plan behind the so-called “Event Horizon Telescope” (the name deserves some discussion; see below) has been to make images of two black holes at the centers of galaxies (the star-cities in which most stars are found.) These are not black holes formed by the collapse of individual stars, such as the ones whose collisions have been observed through their gravitational waves. Central galactic black holes are millions or even billions of times larger! The ones being observed are
- the large and `close’ black hole at the center of the Milky Way (the giant star-city we call home), and
- the immense but much more distant back hole at the center of M87 (a spectacularly big star-megalopolis.)
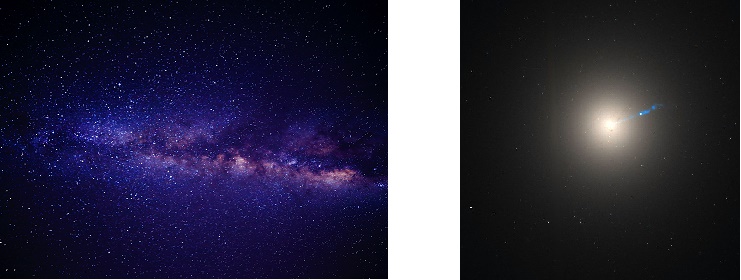
Why go after both of these black holes at the same time? Just as the Sun and Moon appear the same size in our sky because of an accident — the Sun’s greater distance is almost precisely compensated by its greater size — these two black holes appear similarly sized, albeit very tiny, from our vantage point.
Our galaxy’s central black hole has a mass of about four million Suns, and its size is about twenty times wider than our Sun (in contrast to the black holes whose gravitational waves were observed, which are the size of a human city.) But from the center of our galaxy, it takes light tens of thousands of years to reach Earth, and at such a great distance, this big black hole appears as small as would a tiny grain of sand from a plane at cruising altitude. Try to see the sand grains on a beach from a plane window!
Meanwhile, although M87 lies about two thousand times further away, its central black hole has a mass and radius about two thousand times larger than our galaxy’s black hole. Consequently it appears roughly the same size on the sky.
We may get our first images of both black holes at Wednesday’s announcement, though it is possible that so far only one image is ready for public presentation.
How can one see something as black as a black hole?!?
First of all, aren’t black holes black? Doesn’t that mean they don’t emit (or reflect) any light? Yes, and yes. [With a caveat — Hawking radiation — but while that’s very important for extremely small black holes, it’s completely irrelevant for these ones.]
So how can we see something that’s black against a black night sky? Well, a black hole off by itself would indeed be almost invisible. [Though detectable because its gravity bends the light of objects behind it, proving it was a black hole and not a clump of, say, dark matter would be tough.]
But the centers of galaxies have lots of `gas’ — not quite what we call `gas’ in school, and certainly not what we put in our cars. `Gas’ is the generalized term astronomers use for any collection of independently wandering atoms (or ions), atomic nuclei, and electrons; if it’s not just atoms it should really be called plasma, but let’s not worry about that here. Some of this `gas’ is inevitably orbiting the black hole, and some of it is falling in (and, because of complex interactions with magnetic fields, some is being blasted outward before it falls in.) That gas, unlike the black hole, will inevitably glow — it will emit lots of light. What the astronomers are observing isn’t the black hole; they’re observing light from the gas!
And by light, I don’t only mean what we can see with human eyes. The gas emits electromagnetic waves of all frequencies, including not only the visible frequencies but also much higher frequencies, such as those we call X-rays, and much lower frequencies, such as those we call radio waves. To detect these invisible forms of light, astronomers build all sorts of scientific instruments, which we call them `telescopes’ even though they don’t involve looking into a tube as with traditional telescopes.
Is this really a “photograph” of [the gas in the neighborhood of] a black hole?
Yes and (mostly) no. What you’ll be shown is not a picture you could take with a cell-phone camera if you were in a nearby spaceship. It’s not visible light that’s being observed. But it is invisible light — radio waves — and since all light, visible and not, is made from particles called `photons’, technically you could still say is a “photo”-graph.
As I said, the telescope being used in this doesn’t have a set of mirrors in a tube like your friendly neighbor’s amateur telescope. Instead, it uses radio receivers to detect electromagnetic waves that have frequencies above what your traditional radio or cell phone can detect [in the hundred gigahertz range, over a thousand times above what your FM radio is sensitive to.] Though some might call them microwaves, let’s just call them radio waves; it’s just a matter of definition.
So the images you will see are based on the observation of electromagnetic waves at these radio frequencies, but they are turned into something visible for us humans using a computer. That means the color of the image is inserted by the computer user and will be arbitrary, so pay it limited attention. It’s not the color you would see if you were nearby. Scientists will choose a combination of the color and brightness of the image so as to indicate the brightness of the radio waves observed.
If you were nearby and looked toward the black hole, you’d see something else. The gas would probably appear colorless — white-hot — and the shape and brightness, though similar, wouldn’t be identical.
If I had radios for eyes, is this what I would see?
Suppose you had radio receivers for eyes instead of the ones you were born with; is this image what you would see if you looked at the black hole from a nearby planet?
Well, to some extent — but still, not exactly. There’s another very important way that what you will see is not a photograph. It is so difficult to make this measurement that the image you will see is highly processed — that is to say, it will have been cleaned up and adjusted using special mathematics and powerful computers. Various assumptions are key ingredients in this image-processing. Thus, you will not be seeing the `true’ appearance of the [surroundings of the] black hole. You will be seeing the astronomers’ best guess as to this appearance based on partial information and some very intelligent guesswork. Such guesswork may be right, but as we may learn only over time, some of it may not be.
This guesswork is necessary. To make a nice clear image of something so tiny and faint using radio waves, you’d naively need a radio receiver as large as the Earth. A trick astronomers use when looking at distant objects it to build gigantic arrays of large radio receivers, which can be combined together to make a `telescope’ much larger and more powerful than any one receiver. The tricks for doing this efficiently are beyond what I can explain here, but involve the term `interferometry‘. Examples of large radio telescope arrays include ALMA, the center part of which can be seen in this image, which was built high up on a plateau between two volcanoes in the Atacama desert.
But even ALMA isn’t up to the task. And we can’t make a version of ALMA that covers the Earth. So the next best thing is to use ALMA and all of its friends, which are scattered at different locations around the Earth — an incomplete array of single and multiple radio receivers, combined using all the tricks of interferometry. This is a bit like (and not like) using a telescope that is powerful but has large pieces of its lens missing. You can get an image, but it will be badly distorted.
To figure out what you’re seeing, you must use your knowledge of your imperfect lens, and work backwards to figure our what your distorted image really would have looked like if you had a perfect lens.
Even that’s not quite enough: to do this, you need to have a pretty good guess about what you were going to see. That is where you might go astray; if your assumptions are wrong, you might massage the image to look like what you expected instead of how it really ought to look. [Is this a serious risk? I’m not yet expert enough to know the details of how delicate the situation might be.]
It is possible that in coming days and weeks there will be controversies about whether the image-processing techniques used and the assumptions underlying them have created artifacts in the images that really shouldn’t be there, or removed features that should have been there. This could lead to significant disagreements as to how to interpret the images. [Consider these concerns a statement of general prudence based on past experience; I don’t have enough specific expertise here to give a more detailed opinion.]
So in summary, this is not a photograph you’d see with your eyes, and it’s not a complete unadulterated image — it’s a highly-processed image of what a creature with radio eyes might see. The color is arbitrary; some combination of color and brightness will express the intensity of the radio waves, nothing more. Treat the images with proper caution and respect.
Will the image show what [the immediate neighborhood of] a black hole looks like?
Oh, my friends and colleagues! Could we please try to be a little more careful using the phrase `looks like‘? The term has two very different meanings, and in contexts like this one, it really, really matters.
Let me put a spoon into a glass of water: on the left, I’ve drawn a diagram of what it looks like, and on the right, I’ve put a photograph of what it looks like.
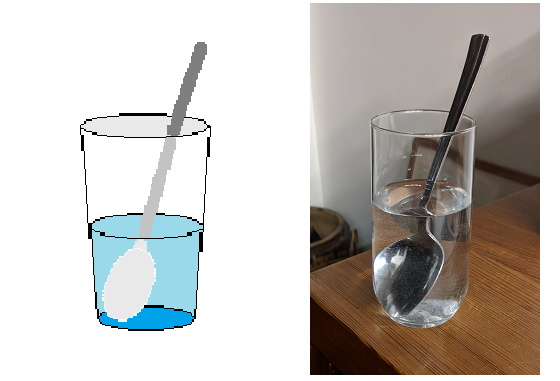
You notice the difference, no doubt. The spoon on the left looks like a spoon; the spoon on the right looks like something invented by a surrealist painter. But it’s just the effect of water and glass on light.
What’s on the left is a drawing of where the spoon is located inside the glass; it shows not what you would see with your eyes, but rather a depiction of the `true location’ of the spoon. On the right is what you will see with your eyes and brain, which is not showing you where the objects are in truth but rather is showing you where the light from those objects is coming from. The truth-depiction is drawn as though the light from the object goes straight from the object into your eyes. But when the light from an object does not take a straight path from the objects to you — as in this case, where the light’s path bends due to interaction of the light with the water and the glass — then the image created in your eyes or camera can significantly differ from a depiction of the truth.
The same issue arises, of course, with any sort of lens or mirror that isn’t flat. A room seen through a curved lens, or through an old, misshapen window pane, can look pretty strange. And gravity? Strong gravity near a black hole drastically modifies the path of any light traveling close by!
In the figure below, the left panel shows a depiction of what we think the region around a black hole typically looks like in truth. There is a spinning disk of gas, called the accretion disk, a sort of holding station for the gas. At any moment, a small fraction of the gas, at the inner edge of the disk, has crept too close to the black hole and is starting to spiral in. There are also usually jets of material flying out, roughly aligned with the axis of the black hole’s rapid rotation and its magnetic field. As I mentioned above, that material is being flung out of the black hole’s neighborhood (not out of the black hole itself, which would be impossible.)
Just as you infer, when you see the broken twisted spoon in the glass, that probably the spoon is not broken or twisted, and the water and glass have refracted the light in familiar ways, so too we must make assumptions to understand what we’re really looking at in truth after we see the images tomorrow.
How serious are these assumptions? Certainly, at their first attempt, astronomers will assume Einstein’s theory of gravity, which predicts how the light is bent around a black hole, is correct. But the details of what we infer from what we’re shown might depend upon whether Einstein’s formulas are precisely right. It also may depend on the accuracy of our understanding of and assumptions about accretion disks. Further complicating the procedure is that the rate and axis of the black hole’s rotation affects the details of the bending of the light, and since we’re not sure of the rotation yet for these black holes, that adds to the assumptions that must gradually be disentangled.
Because of these assumptions, we will not have an unambiguous understanding of the true nature of what appears in these first images.
Are we seeing the `shadow’ of a black hole?
A shadow: that’s what astronomers call it, but as far as I can tell, this word is jargon masquerading as English… the most pernicious type of jargon.
What’s a shadow, in English? Your shadow is a dark area on the ground created by you blocking the light from the Sun, which emits light and illuminates the area around you. How would I see your shadow? I’d look at the ground — not at you.
This is not what we are doing in this case. The gas is glowing, illuminating the region. The black hole is `blocking’ [caution! see below] some of the light. We’re looking straight toward the black hole, and seeing dark areas where illumination doesn’t reach us. This is more like looking at someone’s silhouette, not someone’s shadow!
Still, after you calculate all the bending, you find out that there’s a dark area from which no light emerges, which I’ll informally call a quasi-silhouette. Just outside this is a `photon-sphere’, which creates a bright ring; THIS STATEMENT, MADE IN ALL THE PRESS ARTICLES I COULD FIND, IS MISLEADING, AS ARE THE THREE PARAGRAPHS THAT FOLLOW THE IMAGE BELOW; THEY ARE TRUE FOR A PERFECT CAMERA, BUT NOT FOR A CAMERA THAT CAN BARELY SEE THE IMAGE. BLURRING EFFECTS MAKE THESE CORRECT STATEMENTS SOMEWHAT IRRELEVANT AND CHANGE THE CONCLUSIONS. SEE THIS POST FROM JUNE 2019 THAT EXPLAINS THE SITUATION MORE CLEARLY. That resembles what happens with certain lenses, in contrast to the person’s silhouette shown above, where the light travels in straight lines. Imagine that a human body could bend light in such a way; a whimsical depiction of what that might look like is shown below:
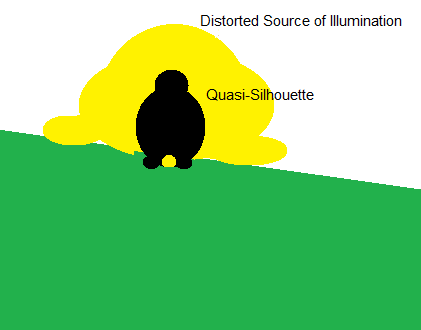
Note also that the black hole’s quasi-silhouette probably won’t be entirely dark. If material from the accretion disk (or a jet pointing toward us) lies between us and the black hole, it can emit light in our direction, partly filling in the dark region.
Thus the quasi-silhouette we’ll see in the images is not the outline of the black hole’s edge, but an effect of the light bending, and is in fact considerably larger than the black hole. In truth it may be as much as 50% larger in radius than the event horizon, and the silhouette as seen in the image may appear more than 2.5 to 5 times larger (depending on how fast the black hole rotates) than the true event horizon — all due to the bent paths of the radio waves. Note Added: THIS ASSUMES THAT THE QUASI-SILHOUETTE IS NOT SUBSTANTIALLY FILLED IN, WHICH, IT TURNS OUT, MAY NOT BE TRUE.
Interestingly, it turns out that the details of how the black hole is rotating don’t much affect the size of the quasi-silhouette. Note Added: THIS IS TRUE, BUT NOT ENTIRELY RELEVANT WHEN USING AN IMPERFECT CAMERA. The black hole in the Milky Way is already understood well enough that astronomers know how big its quasi-silhouette ought to appear, even though we don’t know its rotation speed and axis. The quasi-silhouette’s size in the image will therefore be an important test of Einstein’s formulas for gravity, even on day one. If the size disagrees with expectations, expect a lot of hullabaloo.
What is the event horizon, and is it present in the image?
The event horizon of a black hole, in Einstein’s theory of gravity, is not an object. It’s the edge of a region of no-return, as I’ve explained here. Anything that goes past that point can’t re-emerge, and nothing that happens inside can ever send any light or messages back out.
Despite what some writers are saying, we’re not expecting to see the event horizon in the images. As is hopefully clear by now, what astronomers are observing is (a) the radio waves from the gas near the black hole, after the waves have taken strongly curved paths, and (b) a quasi-silhouette of the black hole from which radio waves don’t emerge. But as I explained above, this quasi-silhouette is considerably larger than the event horizon, both in truth and in appearance. The event horizon does not emit any light, and it sits well inside the quasi-silhouette, not at its edge.
Still, what we’ll be seeing is closer to the event horizon than anything we’ve ever seen before, which is really exciting! And if the silhouette has an unexpected appearance, we just might get our first hint of a breakdown of Einstein’s understanding of event horizons. Don’t bet on it, but you can hope for it.
Can we hope to see the singularity of a black hole?
No, for two reasons.
First, there probably is no singularity in the first place. It drives me nuts when people say there’s a singularity inside a black hole; that’s just wrong. The correct statement is that in Einstein’s theory of gravity, singularities (i.e. unavoidable infinities) arise in the math — in the formulas for black holes (or more precisely, in the solutions to those formulas that describe black holes.) But a singularity in the math does not mean that there’s a singularity in nature! It usually means that the math isn’t quite right — not that anyone made a mistake, but that the formulas that we’re using aren’t appropriate, and need to be modified in some way, because of some natural phenomenon that we don’t yet understand.
A singularity in a formula implies a mystery in nature — not a singularity in nature.
In fact, historically, in every previous case where singularities have appeared in formulas, it simply meant that the formula (or solution) was not accurate in that context, or wasn’t being used correctly. We already know that Einstein’s theory of gravity can’t be complete (it doesn’t accommodate quantum physics, which is also a part of the real world) and it would be no surprise if its incompleteness is responsible for these infinities. The math singularity merely signifies that the physics very deep inside a black hole isn’t understood yet.
[The same issue afflicts the statement that the Big Bang began with a singularity; the solution to the equations has a singularity, yes, but that’s very far from saying that nature’s Big Bang actually began with one.]
Ok, so how about revising the question: is there any hope of seeing the region deep inside the black hole where Einstein’s equations have a singularity? No. Remember what’s being observed is the stuff outside the black hole, and the black hole’s quasi-silhouette. What happens inside a black hole stays inside a black hole. Anything else is inference.
[More precisely, what happens inside a huge black hole stays inside a black hole for eons. For tiny black holes it comes out sooner, but even so it is hopelessly scrambled in the form of `Hawking radiation.’]
Any other questions?
Maybe there are some questions that are bothering readers that I haven’t addressed here? I’m super-busy this afternoon and all day Wednesday with non-physics things, but maybe if you ask the question early enough I can get address it here before the press conference (at 9 am Wednesday, New York City time). Also, if you find my answers confusing, please comment; I can try to further clarify them for later readers.
It’s a historic moment, or at least the first stage in a historic process. To me, as I hope to all of you, it’s all very exciting and astonishing how the surreal weirdness of Einstein’s understanding of gravity, and the creepy mysteries of black holes, have suddenly, in just a few quick years, become undeniably real!
63 Responses
“A singularity in a formula implies a mystery in nature — not a singularity in nature.”
I’m glad someone else sees it that way – I’ve had students and professors roll their eyes with accusation of naivete for suggesting it and vigorously insisting that there is an object of literal, physical, infinite density behind the event horizon.
Thanks for explaining it so beautifully. I really liked the part where you clarified that singularity is in maths and not in nature.
So you exit a high building through stepping out the windows then?
This is the same quality that your trolling science in the other comment is, i.e. none whatsoever. LOL!
”First, there probably is no singularity in the first place. It drives me nuts when people say there’s a singularity inside a black hole; that’s just wrong.”
It’s wrong to say that we know there is a singularity there. But it is equally wrong to say that we know there isn’t. We don’t know.
”The correct statement is that in Einstein’s theory of gravity, singularities (i.e. unavoidable infinities) arise in the math — in the formulas for black holes (or more precisely, in the solutions to those formulas that describe black holes.) But a singularity in the math does not mean that there’s a singularity in nature! It usually means that the math isn’t quite right.”
That’s exactly like saying that the existence of functions like y = 1/x proves that there is something wrong with freshman calculus. There are no singularities in spacetime — GR tells us to excise those regions, precisely because it is a geometric theory. You won’t find talk of “singularities” in textbooks about differential geometry!
”In fact, historically, in every previous case where singularities have appeared in formulas, it simply meant that the formula (or solution) was not accurate in that context, or wasn’t being used correctly. ”
Yes, but GR is unique: being a geometric theory, it teaches you what to do with “singularities”, unlike say Maxwell’s theory.
“We already know that Einstein’s theory of gravity can’t be complete (it doesn’t accommodate quantum physics, which is also a part of the real world)”
No, we don’t ”know” anything of the sort. What we ”know” is that we don’t know how to compute the geometry corresponding to a quantum superposition. The theory is not incomplete, our understanding of it is. And BTW you could equally well say, “We already know that quantum physics can’t be complete (it doesn’t accommodate Einstein’s theory of gravity, which is also a part of the real world)”.
“and it would be no surprise if its incompleteness is responsible for these infinities. ”
Well, it would be no surprise either if the ”singularities” persist in a complete theory.
Look, I agree that there are plenty of good reasons to work on “quantum gravity”, whatever it is. But “WE ALREADY KNOW THAT GR IS WRONG!!!!”
is *not* one of them. Particularly in view of the recent spectacular successes of GR, like this one! I would like to think that these recent successes [such as the direct observation of geodesic deviation, aka gravitational wave detection] will start theorists on the obvious path: take what GR is telling us and *take it seriously*.
I did not say “there is no singularity there” as a statement of fact; I said “there is probably no singularity there”, an opinion I can defend, and therefore my remark was not in violation of your complaint. You put words in my mouth.
A quantum theory of gravity is not general relativity; and if we agree that general relativity must be modified in any way (such as allowing the stress tensor to be an operator instead of a c-number) then we already agree that this cannot be not Einstein’s theory. If the metric or the curvature are operators (or at least not c-numbers) then the infinities we understand geometrically as singularities are automatically modified. Note also that electrodynamics and QCD are obtained in compactifications of general relativity, and in both of them (1) crucial singularities are removed by quantum theory, and (2) the quantum theory is qualitiatively different from the classical theory, to the extent that the classical theory gives very poor intuition in many regimes.
I do know something about this subject; my two most famous papers from the early 2000s involve the resolution of singularities in semi-classical [not classical!] gravity. I am not the world’s expert on quantum gravity, but neither am I uninformed.
If as some believe that space is quantized, than would it be possible that the singularity be the compaction of space or spacetime itself?
I don’t think your question has technical meaning. Physicists have to operate with formulas that are precise, not words that could be interpreted in many different ways.
Why don’t we see the jets that are likely pointing perpendicular to the plane of the accretion disk? as in this recent image:
https://phys.org/news/2019-04-vla-image-key-feature-powerful.html
Are they just too faint (or perhaps even non-existent) that close to the black hole?
Or is there a hint of the jets (very faintly) to the upper left and lower right pf the torus?
I don’t know the answer; the scientists said they were still working on this question and wouldn’t say more. Those faint blurs at the upper left and lower right are tantalizing. However, the jet that aims away from us may be invisible due to Doppler anti-beaming and will also be badly warped. I would therefore expect we might only see the jet pointing toward us. And then? I can only guess: perhaps the jet is too dim to see with the current measurement, or perhaps it only becomes coherent and narrow a little further away from the black hole than the extent of the image.. I’ll have to ask experts this week to try to find this out.
What’s the shape of a black hole? I suspect it’s not really a hole.
A black hole is not an object; it is a region of space (or better, of space time). It is indeed not a hole.
The event horizon — the point of no return — is not a thing, it is a location.
But it has a shape — just as the tropic of Cancer or the Arctic Circle have a shape, though they are locations, not things. .
That shape depends on the details of the black hole’s rotation. A non-rotating black hole has a spherical horizon. A rotating black hole’s event horizon is a slightly flattened sphere. See the “inner horizon” in this figure: https://en.wikipedia.org/wiki/Rotating_black_hole#/media/File:Ergosphere_and_event_horizon_of_a_rotating_black_hole_(no_animation).gif
Thanks for this great post (and the observation is amazing !).
I particularly like that you wrote :
“But a singularity in the math does not mean that there’s a singularity in nature! It usually means that the math isn’t quite right.”
because I repeat the first sentence a lot.
But may I offer a suggestion ? It does not mean the math isn’t quite right (it is a theorem), it means that our mathematical description of nature is not right, that the hypotheses of the theorem has to be changed, that the model fails to describe nature at some scale.
By the way there is a funny example of singularity in Newtonian mechanics:
https://johncarlosbaez.wordpress.com/2016/09/08/struggles-with-the-continuum-part-1/
Good point — I agree my phrasing is ambiguous and your phrasing is clearer than mine.
Taking complex concepts and explaining them simply and elegantly is the hallmark of a superb teacher. Thanks again to Dr. Strassler for making physics accessible to the rest of us.
🙂 You’re too kind…
I’m puzzled by one of the comments in the figure that is in between the one of the spoons and the first of shadow/silhouette diagrams.
The claim is that the Doppler effect causes the left (rotating toward us) side to be brighter, and the right side to be dimmer.
I understand the frequency change due to the Doppler effect, but why is there also a brightness effect?
Apparently this is a relativistic effect known as “Doppler beaming” or the “headlight effect”, in which an isotropic (equal in all directions) emitter beams its light in a cone, as seen by an outside observer, in the direction of the emitter’s motion. The width of the cone (containing all the light emission) narrows, thereby causing increased brightness within the cone, as the emitter’s speed increases, relative to the observer. I think.
The EHT people refer to this in Figure 5 and vicinity of the 5th of their published articles today:
https://iopscience-event-horizon.s3.amazonaws.com/article/10.3847/2041-8213/ab0f43/The_Event_Horizon_Telescope_Collaboration_2019_ApJL_875_L5.pdf
I’ve seen some derivations or attempts at explanation of this effect on the web, but none that I care to recommend. Wolfram has a quasi-derivation (left somewhat to the reader) and a simulation, but it takes a 1-GB download of its cft player.
And belated thanks, Matt, for posting this. It’s the most lucid of all the explanations of the black hole image that I’ve seen.
I’ll try to see if I can come up with a clearer presentation on this effect, but I don’t yet remember all the subtleties myself.
Thanks for the good exposition. Looking at the press release image, I tried to calculate the apparent resolution. This is of course “impossible”
since we do not know the color mapping. I copied the image into Photoshop and used a Gaussdian Blur first to remove noisy JPEG artifacts (diameter 2 px) then another one to just start removing details. If the edge of the visible halo in the image is roughly 240 px in diameter, the needed Gaussian
blur is 8 px diameter. That’s roughly 30 resolution elements.
I’d call that, if correct, quite impressive.
An addition to my resolution comment. I found a different posted image that
somehow had lower contrast, and some outer lobes appeared, at very low
level. Looking at those, and ASSUMING that they are interferometry artifacts, I get a lower number, 10 instead of 30. Comparing the angular extent of the bright blob at left to 360 degrees, I also get about 10 resolution elements across the main doughnut.
From the EHT press release: “VLBI allows the EHT to achieve an angular resolution of 20 micro-arcseconds”. Knowing the distances and sizes, it should be no problem to work out whether 30 or 10 is closer to the right number. I’m afraid I have no time to work it out myself until evening.
Made some calculations this afternoon and found that the ratio of the light ring diameter to the diameter of a Rayleigh diffraction spot is on the order of 3 (!). That would mean there are about 10 total real pixels. Has me a bit concerned. Would appreciate others trying to do this calculation to check…
Matt,
This is a great article for all of us, non scientists.
Thanks a lot!
Kind regards, GEN
Is gravity a real force? Or, are the other forces bending space (distorts it from its equilibrium state thus creating a pseudo force that we have formulated as gravity. So, the mass is the threshold which space is so distorted as to influence the photons. By this definition, the Higgs field is that matrix where the curvature meets that threshold.
Fellow physicist here, who has tried his hand at explaining to a general audience. Wonderful job, will be sharing this link with our students at Roanoke College! Quick question: I guess we’ll know soon, but do you have any idea how many pixels will be in this digital (and processed, as you point out so well) image? I am guessing since it is at the limits of our Earth size radio telescope it may appear a lot grainier than many people are expecting, but am not 100% sure about this. Thanks!
Hi, and thanks. I am not sure, but I believe the image will be so heavily processed that it will not appear grainy; it’s really up to the image processing software used by the scientists which will probably involve a great deal of smoothing. But that’s one way in which it might be misleading; the fact that it’s based on so little information may not be obvious to the eye.
Hello! One of DRobbs students here! Loved this article and how you were able to breakdown what was really going on without getting tangled up in all the technical jargon that a topic like this would normally have. I’ve already gone and shared this page with friends that are not so scientifically literate because I think you’ve done a great job! This only leaves me wondering about the exact influence the programmers had on the program. This article gave the impression that they were straight up manipulating the photo as a photoshop artist would, perhaps that is what you were trying to say to a certain extent. I would think it likely that they used a form of semi or even fully supervised machine learning to reverse engineer lens distortion and make pixel approximations for the smoothing. Of course when you use machine learning that isn’t unsupervised, you still have to consider all kinds of different bias, not just bias on the programmers side but bias from the data too. I definitely intend on looking into this even further!
This is the paper of relevance: https://people.csail.mit.edu/klbouman/pw/papers_and_presentations/cvpr2016_bouman.pdf
Another nice explanation of what you can expect to “see”: https://www.youtube.com/watch?v=zUyH3XhpLTo
Wow, what I could do if I had his budget (low as it is) … 🙂 Yes, it’s very good, especially the use of video and graphics to explain the appearance of the image, though it misses some key points addressed above. In that sense our presentations are nicely complementary.
Hi Matt,
Is there any way to contact you with questions about particle physics? I posted a question on the Of Particular Significance website which appears at the end of the Quantum Fluctuations and Their Energy page, but it has not been answered.
Kind regards,
Julian
I have answered your question on that page. Sorry, but as I’m no longer a paid physicist and I’m writing a book, I’m way behind on answering questions.
Yes, “shadow” is not a precise term for what was to be imaged … but then again the very first paper on the issue almost 20 years ago https://arxiv.org/abs/astro-ph/9912263 has the title “Viewing the Shadow of the Black Hole at the Galactic Center”. And its lead author is the chair of the EHT Science Council, so we may as well use his words …
Oh, we scientists can use whatever word we want when we speak to each other! We can call it the cavalry or the helicoid for all I care. But when we speak to the public, do we want to use the same word? If we use the word “shadow”, no one will understand what we mean, because “shadow” in English means something different. Is that what we want? Or should we try to speak their language, instead of talking to them in our own code?
Thank you Matt. I am really glad there are a few physicists, like yourself who have the rare ability to explain science to the rest of us. I wish I had someone like you as my physics teacher at school.
Thanks for another great blog Matt. It never fails to astound me how such a fractious and belligerent species as homo, – on astronomical scales barely out of chimphood – can muster the brilliance that lets us peer into the inner workings of the universe. And what a treat to have an “explainer” who can articulate these mysteries so well and with such passion.
Keep it up!
Thank you from one of a Non-Experts.
/Herbert identifies two philosophical problems presented by quantum theory—the interpretation question, concerning the physical nature of the reality underlying observation; and the measurement problem, concerning the apparently special role of the measurement act in quantum theory, and various approaches to formally defining the measurement act/.
Mass evaporate, wriggles straightened:
The left spoon is the blue shift of quantum reality at higher energy – the Penrose stairs (wriggles of spacetime) – a dimensionless connect (finite lower bound) ?
In Fynman diagram, electron and positron are the same travel back and forth in TIME. Time dilation differ near Mass and away from mass. So Mass is differ in time, exchanged by photon we observe. But travel in Penrose stairs (wriggles or oscillation) we observe only the blue shift (massless) of bent spoon or quasi silhouette ?
Relativistic Mass m=E/c2=hc/λ
No Ultraviolet catastrophe (termination of geodesic). So no rest mass (GR’s particle), only relativistic mass inferred by neutrino oscillation. This information is not localized ( violation of relativity). So The left spoon’s blue shift does not show the bend ?
0^2 ≠ 0 = unitarity because, there is time difference, massless get angular momentum at speed c. But 0^4 at equivalence principle has time-lapse, appear a relative mass as rest mass. So singularity (0^+n) is a red shift (bent spoon or length contraction) or space expansion (0^-n) ?
Wonderful explanation Matt. Thank you as always. But, if I’m not mistaken, as I sometimes am. You didn’t explain how the massive black hole at the center of a galaxy is created. Would really like to know that! Sue
So would I, and so would all the world’s astronomers. No one is sure… an active area of research.
This is great!
One thing: I think the knowledge moves whatever we will see from the realm of “guess” to that of an “estimate.” There are known bases from which to estimate.
Very nice explanations. Thank you.
You’re most welcome!
So the outcome of the image could be cruxial for old or new physics?
It’s possible. Unlikely (or we’d have probably heard even wilder rumors).
Thank you wonderful explanation, You wrote: ” the surreal weirdness of Einstein’s understanding of gravity ” will probably become true.
If not, what would be the result?
That depends on what the image looks like, no? Newton’s theories worked every time for well over a century… until the 1800s, when they started fraying around the edges, and eventually had to be subsumed into something richer and more complete.
Wonderful explanation of the phenomena to be displayed. Thank you.
Thank you. This is an excellent resource.